Immunotherapeutic Strategies in the Management of Osteosarcoma
Article Information
Rajiv Supra1 and Devendra K Agrawal2*
1College of Osteopathic Medicine, Touro University, Henderson, Nevada
2Department of Translational Research, Western University of Health Sciences, 309 E. Second Street, Pomona, California 91766-1854, USA
*Corresponding Author: Devendra K Agrawal, Department of Translational Research, Western University of Health Sciences, 309 E. Second Street, Pomona, California 91766-1854, USA.
Received: 18 January 2023; Accepted: 01 February 2023; Published: 06 February 2023
Citation:
Rajiv Supra, Devendra K Agrawal. Immunotherapeutic Strategies in the Management of Osteosarcoma. Journal of Orthopedics and Sports Medicine 5 (2023): 32-40
View / Download Pdf Share at FacebookAbstract
Osteosarcoma (OS) is the most common primary malignant bone cancer with a high tendency for metastasis. Although treatment strategies involving surgery and chemotherapy have improved outcomes for patients with OS, the prognosis of recurrent OS is quite unsatisfactory. Primary reasons leading to mortality in OS patients are resistance to currently used therapies and the subsequent lung metastasis. Immunotherapy, however, has been shown to be a promising therapeutic strategy against OS. As research progresses, immunotherapy is gradually becoming irreplaceable. This article provides a critical evaluation of several therapeutic strategies for OS including immunomodulation, vaccine therapy, and immunologic checkpoint blockade.
Keywords
Cancer immunotherapy; Cancer vaccine; Immune checkpoint inhibitors; Immunomodulation; Immunotherapy; Osteosarcoma; Tumor microenvironment; Vaccine therapy
Osteopathic medicine articles Osteopathic medicine Research articles Osteopathic medicine review articles Osteopathic medicine PubMed articles Osteopathic medicine PubMed Central articles Osteopathic medicine 2023 articles Osteopathic medicine 2024 articles Osteopathic medicine Scopus articles Osteopathic medicine impact factor journals Osteopathic medicine Scopus journals Osteopathic medicine PubMed journals Osteopathic medicine medical journals Osteopathic medicine free journals Osteopathic medicine best journals Osteopathic medicine top journals Osteopathic medicine free medical journals Osteopathic medicine famous journals Osteopathic medicine Google Scholar indexed journals Thrombocytopenia articles Thrombocytopenia Research articles Thrombocytopenia review articles Thrombocytopenia PubMed articles Thrombocytopenia PubMed Central articles Thrombocytopenia 2023 articles Thrombocytopenia 2024 articles Thrombocytopenia Scopus articles Thrombocytopenia impact factor journals Thrombocytopenia Scopus journals Thrombocytopenia PubMed journals Thrombocytopenia medical journals Thrombocytopenia free journals Thrombocytopenia best journals Thrombocytopenia top journals Thrombocytopenia free medical journals Thrombocytopenia famous journals Thrombocytopenia Google Scholar indexed journals Osteosarcoma articles Osteosarcoma Research articles Osteosarcoma review articles Osteosarcoma PubMed articles Osteosarcoma PubMed Central articles Osteosarcoma 2023 articles Osteosarcoma 2024 articles Osteosarcoma Scopus articles Osteosarcoma impact factor journals Osteosarcoma Scopus journals Osteosarcoma PubMed journals Osteosarcoma medical journals Osteosarcoma free journals Osteosarcoma best journals Osteosarcoma top journals Osteosarcoma free medical journals Osteosarcoma famous journals Osteosarcoma Google Scholar indexed journals Nephrotoxicity articles Nephrotoxicity Research articles Nephrotoxicity review articles Nephrotoxicity PubMed articles Nephrotoxicity PubMed Central articles Nephrotoxicity 2023 articles Nephrotoxicity 2024 articles Nephrotoxicity Scopus articles Nephrotoxicity impact factor journals Nephrotoxicity Scopus journals Nephrotoxicity PubMed journals Nephrotoxicity medical journals Nephrotoxicity free journals Nephrotoxicity best journals Nephrotoxicity top journals Nephrotoxicity free medical journals Nephrotoxicity famous journals Nephrotoxicity Google Scholar indexed journals Ototoxicity articles Ototoxicity Research articles Ototoxicity review articles Ototoxicity PubMed articles Ototoxicity PubMed Central articles Ototoxicity 2023 articles Ototoxicity 2024 articles Ototoxicity Scopus articles Ototoxicity impact factor journals Ototoxicity Scopus journals Ototoxicity PubMed journals Ototoxicity medical journals Ototoxicity free journals Ototoxicity best journals Ototoxicity top journals Ototoxicity free medical journals Ototoxicity famous journals Ototoxicity Google Scholar indexed journals Mucositis articles Mucositis Research articles Mucositis review articles Mucositis PubMed articles Mucositis PubMed Central articles Mucositis 2023 articles Mucositis 2024 articles Mucositis Scopus articles Mucositis impact factor journals Mucositis Scopus journals Mucositis PubMed journals Mucositis medical journals Mucositis free journals Mucositis best journals Mucositis top journals Mucositis free medical journals Mucositis famous journals Mucositis Google Scholar indexed journals Leukopenia articles Leukopenia Research articles Leukopenia review articles Leukopenia PubMed articles Leukopenia PubMed Central articles Leukopenia 2023 articles Leukopenia 2024 articles Leukopenia Scopus articles Leukopenia impact factor journals Leukopenia Scopus journals Leukopenia PubMed journals Leukopenia medical journals Leukopenia free journals Leukopenia best journals Leukopenia top journals Leukopenia free medical journals Leukopenia famous journals Leukopenia Google Scholar indexed journals Immunotherapeutic articles Immunotherapeutic Research articles Immunotherapeutic review articles Immunotherapeutic PubMed articles Immunotherapeutic PubMed Central articles Immunotherapeutic 2023 articles Immunotherapeutic 2024 articles Immunotherapeutic Scopus articles Immunotherapeutic impact factor journals Immunotherapeutic Scopus journals Immunotherapeutic PubMed journals Immunotherapeutic medical journals Immunotherapeutic free journals Immunotherapeutic best journals Immunotherapeutic top journals Immunotherapeutic free medical journals Immunotherapeutic famous journals Immunotherapeutic Google Scholar indexed journals Natural killer articles Natural killer Research articles Natural killer review articles Natural killer PubMed articles Natural killer PubMed Central articles Natural killer 2023 articles Natural killer 2024 articles Natural killer Scopus articles Natural killer impact factor journals Natural killer Scopus journals Natural killer PubMed journals Natural killer medical journals Natural killer free journals Natural killer best journals Natural killer top journals Natural killer free medical journals Natural killer famous journals Natural killer Google Scholar indexed journals Tumor vaccines articles Tumor vaccines Research articles Tumor vaccines review articles Tumor vaccines PubMed articles Tumor vaccines PubMed Central articles Tumor vaccines 2023 articles Tumor vaccines 2024 articles Tumor vaccines Scopus articles Tumor vaccines impact factor journals Tumor vaccines Scopus journals Tumor vaccines PubMed journals Tumor vaccines medical journals Tumor vaccines free journals Tumor vaccines best journals Tumor vaccines top journals Tumor vaccines free medical journals Tumor vaccines famous journals Tumor vaccines Google Scholar indexed journals Neutrophils articles Neutrophils Research articles Neutrophils review articles Neutrophils PubMed articles Neutrophils PubMed Central articles Neutrophils 2023 articles Neutrophils 2024 articles Neutrophils Scopus articles Neutrophils impact factor journals Neutrophils Scopus journals Neutrophils PubMed journals Neutrophils medical journals Neutrophils free journals Neutrophils best journals Neutrophils top journals Neutrophils free medical journals Neutrophils famous journals Neutrophils Google Scholar indexed journals Eosinophils articles Eosinophils Research articles Eosinophils review articles Eosinophils PubMed articles Eosinophils PubMed Central articles Eosinophils 2023 articles Eosinophils 2024 articles Eosinophils Scopus articles Eosinophils impact factor journals Eosinophils Scopus journals Eosinophils PubMed journals Eosinophils medical journals Eosinophils free journals Eosinophils best journals Eosinophils top journals Eosinophils free medical journals Eosinophils famous journals Eosinophils Google Scholar indexed journals Basophils articles Basophils Research articles Basophils review articles Basophils PubMed articles Basophils PubMed Central articles Basophils 2023 articles Basophils 2024 articles Basophils Scopus articles Basophils impact factor journals Basophils Scopus journals Basophils PubMed journals Basophils medical journals Basophils free journals Basophils best journals Basophils top journals Basophils free medical journals Basophils famous journals Basophils Google Scholar indexed journals T-Regulatory cells articles T-Regulatory cells Research articles T-Regulatory cells review articles T-Regulatory cells PubMed articles T-Regulatory cells PubMed Central articles T-Regulatory cells 2023 articles T-Regulatory cells 2024 articles T-Regulatory cells Scopus articles T-Regulatory cells impact factor journals T-Regulatory cells Scopus journals T-Regulatory cells PubMed journals T-Regulatory cells medical journals T-Regulatory cells free journals T-Regulatory cells best journals T-Regulatory cells top journals T-Regulatory cells free medical journals T-Regulatory cells famous journals T-Regulatory cells Google Scholar indexed journals Osteosarcoma articles Osteosarcoma Research articles Osteosarcoma review articles Osteosarcoma PubMed articles Osteosarcoma PubMed Central articles Osteosarcoma 2023 articles Osteosarcoma 2024 articles Osteosarcoma Scopus articles Osteosarcoma impact factor journals Osteosarcoma Scopus journals Osteosarcoma PubMed journals Osteosarcoma medical journals Osteosarcoma free journals Osteosarcoma best journals Osteosarcoma top journals Osteosarcoma free medical journals Osteosarcoma famous journals Osteosarcoma Google Scholar indexed journals
Article Details
1. Introduction
Osteosarcoma (OS), the most common primary bone tumor, has the highest incidence in adolescents and children with the second highest incidence in adults over the age of 60 [1]. The current clinical treatment involves surgery and chemotherapy that has shown to enhance the 5-year survival rate by >70% in patients with OS, however, it failed in OS patients with metastasis [2]. Additionally, it is more imperative than ever in those subjects with OS who have metastasis to other sites at the time of initial diagnosis [3]. Mainstream chemotherapeutic agents used in the treatment of OS include methotrexate, doxorubicin, ifosfamide, and cisplatin and have extensive side effect profiles. Side effects of these agents such as ototoxicity, mucositis, leukopenia, thrombocytopenia, and nephrotoxicity are problematic and challenging [4-6]. An appropriate command of the immune system using therapeutic agents is essential for tumor control and may provide better outcomes for patients with OS.
In this article, a critical discussion with the emphasis on immunotherapeutic strategies used for treating OS is presented. Strategies include immunomodulation, vaccines, blockage of immunologic checkpoints, and targeted therapy. Immunomodulation consists of activating components of the innate immune system, resulting in upregulating Natural Killer (NK) cells, monocytes, and macrophages, in an attempt to attack tumor cells [7]. Numerous clinical trials have been done on immunotherapies determining the efficacy and application towards treating OS [8,9]. The poor prognosis in patients with OS is a result of early hematogenous spread and resistance to therapy. Additionally, current treatments encounter bottlenecks in chemotherapy resistance and tumor immune escape, which are largely promoted by the microenvironment and tumor stem cells [10,11]. Novel immunotherapeutic strategies consisting of the application of tumor vaccines, genetically modified T cells, immune checkpoint inhibitors, and combination therapies can be used to mitigate treatment side effects, and improve the quality of life in patients with OS [12].
Thus, the focus of the article is on the immunotherapeutic strategies used in the management of OS highlighting the underlying mechanisms of action of immunotherapeutics with a view towards the future where cultivating the strength of these treatments could potentially lead towards a cure for OS.
2.Interaction of Tumor Cells with Immune cells in the Tumor Microenvironment
The immune system consists of an intricate organization of cells that protect and fight against viruses and bacteria. The innate immune system consists of macrophages, NK cells, Dendritic Cells (DCs), neutrophils, eosinophils, and basophils. These cells are the primary defense against foreign pathogens. Mast cells and macrophages start the inflammatory response through various cytokines which interact with other immune cells. Antigen presenting cells, such as DCs, present foreign antigens to be recognized by adaptive immune cells. These adaptive immune cells consist of CD4+ T helper lymphocytes, B lymphocytes, and antigen-specific T lymphocytes. The adaptive and innate immune systems are interconnected and thus work together to eliminate foreign pathogens and remove damaged cells [13,14]. Normally, the adaptive and innate immune systems can detect tumor cells and destroy them through NK cells, secretion of interferon gamma (IFN-g), and activating DCs. However, some cancerous cells evade this response and survive by various mechanisms like downregulating Major Histocompatibility Complex (MHC), recruiting regulatory T cells and myeloid suppressor cells, changing the tumor microenvironment, and upregulating inhibitory ligands and receptors on tumor cells and T cells, respectively [15,16] (Figure 1).
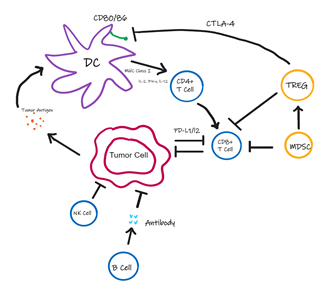
Figure 1: Interaction of Tumor cells with various immune cells. Tumor cells release tumor antigen which is taken up by the Dendritic Cells (DCs) to present to CD4+ T cells followed by the activation of CD8+ T cells. NK cells, B cells, T-Regulatory Cells (TREG) and Myeloid Suppressor Cells (MDSC) in the tumor microenvironment regulate the activation and function of DCs, CD8+ T cells and tumor cell. Expression of regulatory T cells and immune checkpoint proteins allow the tumor to evade the immune response.
3. Macrophages in the Tumor Microenvironment and Novel Therapies
The immune microenvironment in osteosarcoma consists of both adaptive and innate immune cells, specifically T lymphocytes and macrophages [17]. These immune cells can be found in the adjacent lymphoid structures or in the core of the tumor [18]. OS cells are able to modulate both immune systems and therefore induce an immune-tolerant state which is conducive of metastasizing tumor cells [19]. OS patients that have higher immune scores with increased immune cells infiltrating the microenvironment have been documented to have a better prognosis than OS patients with lower immune scores [20]. Hence, the cross talk with tumor cells and the tumor immune microenvironment may be indispensable to further study and research for developing immunotherapies. Furthermore, macrophages are at a higher proportion of immune cells when compared to other immune cells in the tumor microenvironment of OS [21]. This reinforces the high plasticity of macrophages especially when presenting with inverse phenotypes and activating signals. For example, the pro-inflammatory phenotype of macrophages M1 and the anti-inflammatory phenotype M2, both serve different purposes depending on the immune response. When the immune system triggers tumor suppression, M1 macrophages serve as the major defense cells and cytokines such as IL-12, IL-6, and IL-1 are secreted [22-24]. In contrast, immune suppression is associated with the M2 macrophage response leading to tumor metastasis. Research has revealed that a higher proportion of tumor associated macrophages correlated with worse prognosis in most solid cancers and the M2 related cytokines showed increased events of lung metastasis in OS [25-27]. Interestingly, shifting the macrophage response from the M2 to M1 phenotype decreased incidence of lung metastasis in OS, further strengthening the role macrophages play in the tumor microenvironment [28]. However, controversy remains whether macrophages are pro-tumor or anti-tumor in the OS microenvironment. Previous research showed increased infiltration of macrophage in OS tumor cells, decreased rates of metastasis, and increased overall survival outcome, whereas M2 macrophage infiltration was associated with a poor prognosis [29,30]. Therefore, recent therapies have attempted to influence macrophage response within the tumor microenvironment. For example, All-Trans Retinoic Acid (ATRA) was proven to reduce pulmonary metastasis in OS through potentially indirectly deviating macrophages from M2 polarization [31,32]. Moreover, naturally occurring substances such as dihydroxycoumarins were also studied to induce macrophage polarization from M2 to M1 phenotype in OS treatments [33]. Dihydroxycoumadins inhibit the production of growth factors like TGF-b and cytokines such as IL-10. They also reduce the phosphorylation of STAT3 during M2 polarization, interfering with its activation [33,34]. Zoledronic acid, another potential therapeutic for OS, has been shown to interfere with M2 polarization causing macrophages in the tumor microenvironment to polarize back to the M1 phenotypes [35]. In a randomized study using zoledronate, however, the treatment using this agent did not result in improved outcomes in patients with OS [36]. Similarly, another study revealed zoledronate may induce bipotent macrophages by deleteriously polarizing to CD68+/CD163+ [37]. CD163 staining is associated with higher cMaf expression (a transcription factor associated with M2 macrophage polarization) while CD68 is stated to be an M1-polarized macrophage marker [38]. Therefore, the therapeutic potential of zoledronate still needs future research to elucidate whether it has a positive or negative effect on OS. Another novel immunomodulatory drug that increases inflammatory cytokines and triggers macrophage polarization is Mifamurtide [39]. Studies have demonstrated the potential of mifamurtide as an anti-tumor agent not only by restricting M2 initiation via decreased Akt phosphorylation and decreasing STAT3 levels, but also by shifting macrophage polarization to the intermediate phenotype M1-M2 [40]. Macrophage polarization has been a promising target for novel therapies in modulating the progression of OS.
4. Immune Checkpoint Inhibitors
Recent research has focused on immune checkpoint inhibitors and their association with the inhibition of tumor progression. Immune checkpoints enable immune tolerance against cancer in addition to preventing autoimmune disorders. Programmed death receptor-1 (PD-1) and CTLA-4 are the main inhibitory receptors on T cells and have been major candidates as targets for novel therapies against OS [41]. Normally, activated T cells express PD-1 on the surface and suppress the immune response through PD-L1, the ligand for PD-1, that is usually expressed on tumor tissues. Studies showed that anti-PD-1 therapy redirecting M2 macrophages to M1 resulted in regression of lung metastasis in a murine model of OS [42]. The efficacy of nivolumab in a murine model of OS was investigated and revealed nivolumab treated mice had significantly fewer rates of metastatic lung lesions, however, primary tumor volume and growth were not affected [43]. Another study revealed high levels of PD-L1 in OS patients and expression of PD-L1 was positively correlated with Tumor-Infiltrating Lymphocytes (TILs). The overall survival time of patients with low PD-L1 expression was 89 months and those with high levels of PD-L1 had a median survival duration of 28 months [44]. Hingorani et al. [45] revealed increased expression of immunosuppressive monocytes and increased CTLA-4 in T cells in patients with pediatric sarcoma. High levels of PD-1 expression was also noted on peripheral CD8+ and CD4+ T cells in OS patients and CD4+ T cells in patients with metastasis had significantly higher expression levels of PD-1 [46]. Moreover, combination therapy for OS has shown promising results using anti-CTLA-4 and anti-PD L1 antibodies which showed improved overall survival in a murine model with OS, whereas no benefits were noted when treated with anti-CTLA-4 antibody alone [47]. Although the therapeutic effects of combination immune checkpoint inhibitors have not been confirmed in clinical trials, there have been several reports that immunotherapies with nivolumab plus ipilimumab showed considerable tumor remission in metastatic OS patients [48,49]. Immunotherapies, however, do not come without adverse effects. Immune check point inhibitors like anti-PD-1 inhibitors are antigen agnostic which results in severe immune hyperactivation. This cytokine storm can result in encephalopathy, coagulation disorders, cerebrovascular events, and cardiovascular related events. The toxicities from these therapies can be neutralized by using steroids but impairs the effectiveness of the immunotherapies as a result. Studies showed concomitant medication of PD-1/PD-L1 immune check point therapies with prednisone can worsen the clinical outcomes in patients with OS [50,51]. Tocilizumab, an antibody that targets IL-6, was shown to successfully reduce adverse reactions from immunotherapies in a wide variety of models [52]. This reinforces the fact that antagonists of cytokine receptors in addition to immune check point inhibitor therapy can be a therapeutic option to help better manage OS in the future.
5. Adoptive T-Cell Therapy
Active and adoptive immunotherapies are used in the treatment of OS. Active immunotherapies using DCs, pulsed vaccines, and cytokines influence immune responses towards tumor cells. Adoptive immunotherapy involves administering ex vivo-expanded tumor specific cytotoxic immune cells [53]. This process includes harvesting autologous immune cells, expanding them using ex vivo cultures with modified T cell receptors or Chimeric Antigen Receptors (CAR) for adoptive therapy [54,55].
Tumor Associated Antigens (TAA) are recognized by engineered CAR T cells. The CAR includes an ectodomain consisting of a single chain variable fragment of an antibody which is able to identify TAAs. The endodomain is derived from CD3 zeta chain involved in intracellular signaling. The structure as a whole allows T cells to identify TAAs and result in tumor cytotoxicity in an MHC independent pathway [56]. HER2 has been shown to be expressed by OS cells in 40-60% of primary OS samples and the safety of HER2 CAR-T cells have been researched without dose limiting toxic reactions [57,58]. Interestingly, even with low levels of HER2 expression, CAR-T cells have been shown to effectively target HER2 which reinforces the potential CAR-Ts have to subvert cancerous cells. A recent study showed metastatic tumors that were not sensitive to chemotherapy were effectively depleted by CAR-Ts engineered on IL-11 receptor alpha chain and HER2 in OS murine models [57,59]. Additionally, CAR-T cells have been shown to inhibit Insulin-Like Growth Factor 1 (IGF-1) and receptor tyrosine and prolong the survival in OS mouse models [60]. The limitations using CAR-T include its side effects such as encephalopathies, end organ damage, and systemic inflammatory syndromes [61,62]. Future studies on CAR-T cell therapy will require addressing the drawbacks to this therapy and limiting the side effects in patients with OS.
Superior to CARs in targeting efficacy is the T Cell Receptors (TCRs) because they are able to interact with both surface antigenic peptides presented by HLA and tumors intracellularly [63]. This feature of TCRs allows for more advanced applications in solid tumors. Germline antigens from cancer cells are expressed in a reduced manner in tissues from different histologic origins. The germ cells lack MHC expression and are therefore protected from TCR T cell immunotherapy [64]. A specific cancer germline antigen, NY-ESO-1, is found in 31% of OS tumors and TCR therapy targeting this antigen is currently under investigation. Recent research revealed that the engineered CD8+ T cells can encode MHC-I restricted TCRs for therapy. Lu et al. [65] used CD4+ T cells transduced with MHC-II-restricted TCRs in patients with OS which resulted in objective partial responses in patients with metastatic lesions of the lung.
6. Cancer Vaccine Therapies
Cancer vaccines have been studied and implemented to have anti-tumor effects by stimulating the immune response in patients. Peptides, DNA, RNA, and tumor antigens have been presented on cells to initiate the immune system [66]. Marcove et al. [67] initiated the use of autologous tumor lysates as vaccine therapies for cancer and resulted in increased survival rates in OS patients. Cancer vaccines have been classified into non-cell based, autologous, and tumor cell vaccines. Innate immune cells such as DCs, T cells, and macrophages are exploited using the immune cell vaccines. However, there are limitations to this mode of therapy mainly due to the immunosuppressive molecules within the tumor environment. The most widely used vaccination approach is through using DCs. These professional antigen presenting cells endocytose and present to naïve T cells that ultimately differentiate into tumor killing cells [68]. DC vaccines are able to reduce immunosuppression caused by cancerous cells and the latest FDA approved drug, ilixadencel, has shown great success [69]. The process of engineering DC vaccines first involves isolating peripheral mononuclear cells that are then pulsed with tumor antigen ex vivo. These cells are then injected back into the patient (Figure 2) [68]. DC vaccines can be classified based on the type of pulsed antigens for example, DCs can be co-cultured with tumor specific proteins, transfected with DNA coding for tumor antigens, or tumor lysates [69]. Studies have shown promising clinical responses with DCs pulsed with peptides from specific cancer germline antigens against OS [70]. Furthermore, combinational therapy using DCs and drugs targeting TGF-b suppresses the rates of metastasis through remodeling the tumor microenvironment [71]. CD14+ monocytes and CD34+ have been the main source of DCs in clinical trials in humans, however, Zhou et al. [72] affirmed that conventional type 1 dendritic cells can elicit tumor-specific T cell cytotoxicity in a murine OS model. This finding advances the horizon for using and developing DC vaccines for OS.

Figure 2: Process of engineering ex vivo DC vaccines. Progenitor cells are derived from patients and loaded with tumor antigens which subsequently active cytotoxic T cells to kill cancer cells.
In addition to DCs, macrophages and γδ T cells have been used in tumor vaccines in the form of Chimeric Antigen Receptor Macrophages (CAR-Ms) and peptide-pulsed γδ T cells. CD8+ T cells are primed by the γδ T cells [73]. The γδ T cells have been reported to be superior to DC vaccines due to its HLA-independent manner in activating cytotoxic activity towards cancer cells [68]. Research revealed that γδ T cells can attack OS cells despite the limited susceptibility of cancer cells towards γδ T cell toxicity [74]. Rapamycin, an mTOR inhibitor can magnify the γδ T cell response by increasing tumor penetration [75]. Additionally, researchers recently manufactured novel CAR-Ms in order to phagocytose tumor cells, promote a pro-inflammatory tumor microenvironment through M2 to M1 polarization, and presenting antigens to naïve T cells subsequently influencing them to turn into cytotoxic T cells. An engineered anti-HER2 CAR-M induced a significant decrease in tumor burden and increased survival in murine models with metastatic lung cancer [76]. Polymer nanocarriers were fabricated in order to deliver genes encoding interferon-γ and CAR to macrophages in vivo and was able to code tumor specific CAR expressing macrophages in situ [77]. Collectively, these studies have potential to further our understanding of using γδ T cell and CAR- M vaccines against OS.
Autologous tumor vaccines are beneficial in that they bypass the culture ex vivo process and DC isolation and instead directly influence the DC response in vivo. As tumor cells are isolated from patients, they are expanded and irradiated before being re-infused [78]. A recent study revealed a combined autologous tumor vaccine with IL-2 together in canines with OS and resulted in prolonged survival compared to those that received amputation [79]. The safety and efficacy of single or combined autologous tumor cell vaccines has yet to be elucidated in human subjects. Viral and peptide vaccines have similar mechanisms of action by presenting the antigen to DCs in vivo. The tumor associated antigen Papilloma Binding Factor (PBF) derived peptide vaccine has long been researched for HLA-A2/A24+ OS patients. PBF is a transcription factor whose levels have been shown to reach up to 92% in OS patients. Peptides from PBF A24.2 are shown to activate cytotoxic T cells in OS patients positive with HLA-A24, and therefore can trigger the immune response to eliminate cancer cells [80]. A study was performed in creating a peptide tetramer targeting LSA and QVT peptides which showed toxicity against HLA-A11+PBF+ cells from OS [81]. Other vaccines such as those based on HER2 have been underappreciated by benefits seen in OS canine models. The recombinant listeria vaccine that targeted HER2 showed reduced levels of metastasis and improved prognosis when compared to the control group [82].
7. Cytokines
Studies revealed that cytokines can influence the immune response by interacting with immune therapeutic cells and other inflammatory cytokines such as IL-8, TNF-a which are correlated with the progression of OS [83]. Potentially the cytokine-based approaches have been the most widely used in immunotherapeutics and have been used for cancer therapy for over three decades. Their ability to activate T cells and modulate antigen presentation makes it a viable therapy option [53]. Macrophages release cytokines which then engage in then engage in the inflammatory response during cancerous states. IL-12, an immune-positive regulating cytokine can promote B and T cell differentiation, proliferation, and antibody formation. It has an integral role in reducing gastrointestinal bleeding caused by chemotherapy and improving patient tolerance to cancer radiotherapy. A study revealed that tumor cells from high grade OS patients were lysed by IL-15 induced NK cells [84]. In primary metastatic OS patients who were administered IL-2, a survival rate of more than 40% was documented at 3 years [85]. Limitations, however, exist with using cytokines due to their toxic side effect profile in patients with OS from administering a sufficient dose to activate the immune system [86].
8. Cytokines
Treatments for OS are transitioning from the standard therapies of chemotherapy and surgery towards more novel approaches such as immunotherapy. With improved understanding of the immune response to OS, many patients will benefit from immunotherapies for years to come. However, to date, new immunotherapeutic drugs have been limited in the use for OS. The main obstacle being limited T cell infiltration and immune hyperresponsiveness. Additionally, the tumor microenvironment makes these therapies difficult to penetrate into and kill cancer cells. Generally, immunotherapy shows promising data for treating OS, however further research is needed to determine more precise curative effects.
Author Contributions:
Concept and design: RS, DKA; Literature Search: RS, DKA; Critical review and interpretation of the findings: RS, DKA; Drafting the article: RS; Revising and editing the manuscript: RS, DKA; Final approval of the article: RS, DKA.
Funding:
The research work of DKA is supported by the research grants R01 HL144125 and R01HL147662 from the National Heart, Lung, and Blood Institute, National Institutes of Health, USA. The contents of this article are solely the responsibility of the authors and do not necessarily represent the official views of the National Institutes of Health.
Institutional Review Board Statement:
Not applicable.
Informed Consent Statement:
Not applicable
Data Availability Statement:
Not applicable since the information is gathered from published articles.
Acknowledgments:
None
Conflicts of Interest:
The authors declare no conflict of interest.
References
- Gill J, Ahluwalia MK, Geller D, et al. New targets and approaches in osteosarcoma. Pharmacol Ther 137 (2013): 89-99.
- Kansara M, Teng MW, Smyth MJ, et al. Translational biology of osteosarcoma. Nat Rev Cancer 14 (2014): 722-735.
- Zhao J, Dean DC, Hornicek FJ, et al. Emerging next-generation sequencing-based discoveries for targeted osteosarcoma therapy. Cancer Lett 474 (2020): 158-167.
- Smrke A, Anderson PM, Gulia A, et al. Future Directions in the Treatment of Osteosarcoma. Cells. 10 (2021): 172.
- Meyers PA, Healey JH, Chou AJ, et al. Addition of pamidronate to chemotherapy for the treatment of osteosarcoma. Cancer 117 (2011): 1736-1744.
- Zhang B, Zhang Y, Li R, et al. The efficacy and safety comparison of first-line chemotherapeutic agents (high-dose methotrexate, doxorubicin, cisplatin, and ifosfamide) for osteosarcoma: a network meta-analysis. J Orthop Surg Res 15 (2020): 51.
- Loeb DM. Is there a role for immunotherapy in osteosarcoma? Cancer Treat Res 152 (2009): 447-457.
- Wedekind MF, Wagner LM, Cripe TP. Immunotherapy for osteosarcoma: Where do we go from here? Pediatr Blood Cancer 65 (2018): e27227.
- Yoshida K, Okamoto M, Sasaki J, et al. Anti-PD-1 antibody decreases tumour-infiltrating regulatory T cells. BMC Cancer 20 (2020): 25.
- Brown HK, Tellez-Gabriel M, Heymann D. Cancer stem cells in osteosarcoma. Cancer Lett 386 (2017): 189-195.
- Alfranca A, Martinez-Cruzado L, Tornin J, et al. Bone microenvironment signals in osteosarcoma development. Cellular and Molecular Life Sciences 72 (2015): 3097-3113.
- Burgess C, Hammond J. Modernisation of the spectroscopic General Chapters in the United States Pharmacopeia (USP). Spectroscopy Europe 26 (2015).
- de Visser KE, Eichten A, Coussens LM. Paradoxical roles of the immune system during cancer development. Nat Rev Cancer 6 (2006): 24-37.
- Mackall CL, Merchant MS, Fry TJ. Immune-based therapies for childhood cancer. Nat Rev Clin Oncol 11 (2014): 693-703.
- Nishikawa H, Sakaguchi S. Regulatory T cells in cancer immunotherapy. Curr Opin Immunol 27 (2014): 1-7.
- Diaz-Montero CM, Finke J, Montero AJ. Myeloid-Derived Suppressor Cells in Cancer: Therapeutic, Predictive, and Prognostic Implications. Semin Oncol 41 (2014): 174-184.
- Mantovani A, Allavena P, Sica A, et al. Cancer-related inflammation. Nature 454 (2008): 436-444.
- Belli C, Trapani D, Viale G, et al. Targeting the microenvironment in solid tumors. Cancer Treat Rev 65 (2018): 22-32.
- Anwar MA, El-Baba C, Elnaggar MH, et al. Novel therapeutic strategies for spinal osteosarcomas. Semin Cancer Biol 64 (2020): 83-92.
- Zhang C, Zheng JH, Lin ZH, et al. Profiles of immune cell infiltration and immune-related genes in the tumor microenvironment of osteosarcoma. Aging 12 (2020): 3486-3501.
- Yu Y, Zhang H, Ren T, et al. Development of a prognostic gene signature based on an immunogenomic infiltration analysis of osteosarcoma. J Cell Mol Med 24 (2020): 11230-11242.
- Mirabello L, Zhu B, Koster R, et al. Frequency of Pathogenic Germline Variants in Cancer-Susceptibility Genes in Patients with Osteosarcoma. JAMA Oncol 6 (2020): 724.
- Mosser DM, Edwards JP. Exploring the full spectrum of macrophage activation. Nat Rev Immunol 8 (2008): 958-969.
- Sica A, Mantovani A. Macrophage plasticity and polarization: in vivo veritas. Journal of Clinical Investigation 122 (2012): 787-795.
- Wolf-Dennen K, Gordon N, Kleinerman ES. Exosomal communication by metastatic osteosarcoma cells modulates alveolar macrophages to an M2 tumor-promoting phenotype and inhibits tumoricidal functions. Oncoimmunology 9 (2020).
- Han Y, Guo W, Ren T, et al. Tumor-associated macrophages promote lung metastasis and induce epithelial-mesenchymal transition in osteosarcoma by activating the COX-2/STAT3 axis. Cancer Lett 440-441 (2019): 116-125.
- Yang L, Zhang Y. Tumor-associated macrophages: from basic research to clinical application. J Hematol Oncol 10 (2017): 58.
- Dhupkar P, Gordon N, Stewart J, et al. Anti-PD-1 therapy redirects macrophages from an M2 to an M1 phenotype inducing regression of OS lung metastases. Cancer Med 7 (2018): 2654-2664.
- Heymann MF, Lézot F, Heymann D. The contribution of immune infiltrates and the local microenvironment in the pathogenesis of osteosarcoma. Cell Immunol 343 (2019): 103711.
- Buddingh EP, Kuijjer ML, Duim RAJ, et al. Tumor-Infiltrating Macrophages Are Associated with Metastasis Suppression in High-Grade Osteosarcoma: A Rationale for Treatment with Macrophage Activating Agents. Clinical Cancer Research 17 (2011): 2110-2119.
- Xue-Jing S, Sen-Feng X, Ying-Qian , et al. Inhibition of M2-like macrophages by all-trans retinoic acid prevents cancer initiation and stemness in osteosarcoma cells. Acta Pharmacol Sin 40 (2019): 1343-1350.
- Zhou Q, Xian M, Xiang S, et al. All-Trans Retinoic Acid Prevents Osteosarcoma Metastasis by Inhibiting M2 Polarization of Tumor-Associated Macrophages. Cancer Immunol Res 5 (2017): 547-559.
- Kimura Y, Sumiyoshi M. Antitumor and antimetastatic actions of dihydroxycoumarins (esculetin or fraxetin) through the inhibition of M2 macrophage differentiation in tumor-associated macrophages and/or G1 arrest in tumor cells. Eur J Pharmacol 746 (2015): 115-125.
- Fukuda H, Nakamura S, Chisaki Y, et al. Daphnetin inhibits invasion and migration of LM8 murine osteosarcoma cells by decreasing RhoA and Cdc42 expression. Biochem Biophys Res Commun 471 (2016): 63-67.
- Tsagozis P, Eriksson F, Pisa P. Zoledronic acid modulates antitumoral responses of prostate cancer-tumor associated macrophages. Cancer Immunology, Immunotherapy 57 (2008): 1451-1459.
- Gomez-Brouchet A, Illac C, Gilhodes J, et al. CD163-positive tumor-associated macrophages and CD8-positive cytotoxic lymphocytes are powerful diagnostic markers for the therapeutic stratification of osteosarcoma patients: An immunohistochemical analysis of the biopsies from the French OS2006 phase 3 trial. Oncoimmunology 6 (2017): e1331193.
- Gomez-Brouchet A, Gilhodes J, van Acker N, et al. Characterization of Macrophages and Osteoclasts in the Osteosarcoma Tumor Microenvironment at Diagnosis: New Perspective for Osteosarcoma Treatment? Cancers (Basel) 13 (2021): 423.
- Barros MHM, Hauck F, Dreyer JH, et al. Macrophage Polarisation: an Immunohistochemical Approach for Identifying M1 and M2 Macrophages. PLoS One 8 (2013): e80908.
- Múdry P, Kýr M, Rohleder O, et al. Improved osteosarcoma survival with addition of mifamurtide to conventional chemotherapy – Observational prospective single institution analysis. J Bone Oncol 28 (2021): 100362.
- Punzo F, Bellini G, Tortora C, et al. Mifamurtide and TAM-like macrophages: effect on proliferation, migration and differentiation of osteosarcoma cells. Oncotarget 11 (2020): 687-698.
- Conforti F, Pala L, Bagnardi V, et al. Cancer immunotherapy efficacy and patients’ sex: a systematic review and meta-analysis. Lancet Oncol 19 (2018): 737-746.
- Dhupkar P, Gordon N, Stewart J, et al. Anti-PD-1 therapy redirects macrophages from an M2 to an M1 phenotype inducing regression of OS lung metastases. Cancer Med 7 (2018): 2654-2564.
- Zheng B, Ren T, Huang Y, et al. PD-1 axis expression in musculoskeletal tumors and antitumor effect of nivolumab in osteosarcoma model of humanized mouse. J Hematol Oncol 11 (2018): 16.
- Shen JK, Cote GM, Choy E, et al. Programmed Cell Death Ligand 1 Expression in Osteosarcoma. Cancer Immunol Res 2 (2014): 690-698.
- Hingorani P, Maas ML, Gustafson MP, et al. Increased CTLA-4+ T cells and an increased ratio of monocytes with loss of class II (CD14+ HLA-DRlo/neg) found in aggressive pediatric sarcoma patients. J Immunother Cancer 3 (2015): 35.
- Zheng W, Xiao H, Liu H, et al. Expression of programmed death 1 is correlated with progression of osteosarcoma. APMIS 123 (2015): 102-107.
- Lussier DM, Johnson JL, Hingorani P, et al. Combination immunotherapy with α-CTLA-4 and α-PD-L1 antibody blockade prevents immune escape and leads to complete control of metastatic osteosarcoma. J Immunother Cancer 3 (2015): 21.
- Nuytemans L, Sys G, Creytens D, et al. NGS-analysis to the rescue: dual checkpoint inhibition in metastatic osteosarcoma – a case report and review of the literature. Acta Clin Belg 76 (2021): 162-167.
- Sterz U, Grube M, Herr W, et al. Case Report: Dual Checkpoint Inhibition in Advanced Metastatic Osteosarcoma Results in Remission of All Tumor Manifestations—A Report of a Stunning Success in a 37-Year-Old Patient. Front Oncol 11 (2021).
- Cortellini A, Tucci M, Adamo V, et al. Integrated analysis of concomitant medications and oncological outcomes from PD-1/PD-L1 checkpoint inhibitors in clinical practice. J Immunother Cancer 8 (2020): e001361.
- Haanen JBAG, Carbonnel F, Robert C, et al. Management of toxicities from immunotherapy: ESMO Clinical Practice Guidelines for diagnosis, treatment and follow-up. Annals of Oncology 28 (2017): 19-42.
- Stroud CR, Hegde A, Cherry C, et al. Tocilizumab for the management of immune mediated adverse events secondary to PD-1 blockade. Journal of Oncology Pharmacy Practice 25 (2019): 551-557.
- Mori K, Rédini F, Gouin F, et al. Osteosarcoma: current status of immunotherapy and future trends (Review). Oncol Rep 15 (2006): 693-700.
- Rosenberg SA, Restifo NP. Adoptive cell transfer as personalized immunotherapy for human cancer. Science (1979) 348 (2015): 62-68.
- Yee C, Lizee GA. Personalized Therapy. The Cancer Journal 23 (2017): 144-148.
- Balta E, Wabnitz GH, Samstag Y. Hijacked Immune Cells in the Tumor Microenvironment: Molecular Mechanisms of Immunosuppression and Cues to Improve T Cell-Based Immunotherapy of Solid Tumors. Int J Mol Sci 22 (2021): 5736.
- Ahmed N, Salsman VS, Yvon E, et al. Immunotherapy for Osteosarcoma: Genetic Modification of T cells Overcomes Low Levels of Tumor Antigen Expression. Molecular Therapy 17 (2009): 1779-1787.
- O’Rourke DM, Nasrallah MP, Desai A, et al. A single dose of peripherally infused EGFRvIII-directed CAR T cells mediates antigen loss and induces adaptive resistance in patients with recurrent glioblastoma. Sci Transl Med 9 (2017).
- Huang G, Yu L, Cooper LJN, et al. Genetically Modified T cells Targeting Interleukin-11 Receptor α-Chain Kill Human Osteosarcoma Cells and Induce the Regression of Established Osteosarcoma Lung Metastases. Cancer Res 72 (2012): 271-281.
- Huang X, Park H, Greene J, et al. IGF1R- and ROR1-Specific CAR T Cells as a Potential Therapy for High Risk Sarcomas. PLoS One 10 (2015): e0133152.
- Gust J, Hay KA, Hanafi LA, et al. Endothelial Activation and Blood–Brain Barrier Disruption in Neurotoxicity after Adoptive Immunotherapy with CD19 CAR-T Cells. Cancer Discov 7 (2017): 1404-1419.
- Théoleyre S, Mori K, Cherrier B, et al. Phenotypic and functional analysis of lymphocytes infiltrating osteolytic tumors: use as a possible therapeutic approach of osteosarcoma. BMC Cancer 5 (2005): 123.
- Brameshuber M, Kellner F, Rossboth BK, et al. Monomeric TCRs drive T cell antigen recognition. Nat Immunol 19 (2018): 487-496.
- Iura K, Kohashi K, Ishii T, et al. MAGEA4 expression in bone and soft tissue tumors: its utility as a target for immunotherapy and diagnostic marker combined with NY-ESO-1. Virchows Archiv 471 (2017): 383-392.
- Lu YC, Parker LL, Lu T, et al. Treatment of Patients With Metastatic Cancer Using a Major Histocompatibility Complex Class II–Restricted T-Cell Receptor Targeting the Cancer Germline Antigen MAGE-A3. Journal of Clinical Oncology 35 (2017): 3322-3329.
- Pollack SM, Ingham M, Spraker MB, et al. Emerging Targeted and Immune-Based Therapies in Sarcoma. Journal of Clinical Oncology 36 (2018): 125-135.
- Marcove RC, Mike V, Huvos AG, et al. Vaccine Trials for Osteogenic Sarcoma: A Preliminary Report. CA Cancer J Clin 23 (1973): 74-80.
- Wang Z, Wang Z, Li B, et al. Innate Immune Cells: A Potential and Promising Cell Population for Treating Osteosarcoma. Front Immunol 10 (2019).
- Rizell M, Sternby Eilard M, Andersson M, et al. Phase 1 Trial With the Cell-Based Immune Primer Ilixadencel, Alone, and Combined With Sorafenib, in Advanced Hepatocellular Carcinoma. Front Oncol 9 (2019).
- Krishnadas DK, Shusterman S, Bai F, et al. A phase I trial combining decitabine/dendritic cell vaccine targeting MAGE-A1, MAGE-A3 and NY-ESO-1 for children with relapsed or therapy-refractory neuroblastoma and sarcoma. Cancer Immunology, Immunotherapy 64 (2015): 1251-1260.
- Kawano M, Tanaka K, Itonaga I, et al. Dendritic cells combined with anti-GITR antibody produce antitumor effects in osteosarcoma. Oncol Rep 34 (2015): 1995-2001.
- Zhou Y, Slone N, Chrisikos TT, et al. Vaccine efficacy against primary and metastatic cancer with in vitro-generated CD103 + conventional dendritic cells. J Immunother Cancer 8 (2020): e000474.
- Altvater B, Pscherer S, Landmeier S, et al. Activated human γδ T cells induce peptide-specific CD8+ T-cell responses to tumor-associated self-antigens. Cancer Immunology, Immunotherapy 61 (2012): 385-396.
- Kato Y, Tanaka Y, Miyagawa F, et al. Targeting of Tumor Cells for Human γδ T Cells by Nonpeptide Antigens. The Journal of Immunology 167 (2001): 5092-5098.
- Cao G, Wang Q, Li G, et al. mTOR inhibition potentiates cytotoxicity of Vγ4 γδ T cells via up-regulating NKG2D and TNF-α. J Leukoc Biol 100 (2016): 1181-1189.
- Klichinsky M, Ruella M, Shestova O, et al. Human chimeric antigen receptor macrophages for cancer immunotherapy. Nat Biotechnol 38 (2020): 947-953.
- Kang M, Lee SH, Kwon M, et al. Nanocomplex?Mediated In Vivo Programming to Chimeric Antigen Receptor?M1 Macrophages for Cancer Therapy. Advanced Materials 33 (2021): 2103258.
- Dyson KA, Stover BD, Grippin A, et al. Emerging trends in immunotherapy for pediatric sarcomas. J Hematol Oncol 12 (2019): 78.
- Flesner BK, Wood GW, Gayheart?Walsten P, et al. Autologous cancer cell vaccination, adoptive T ?cell transfer, and interleukin?2 administration results in long?term survival for companion dogs with osteosarcoma. J Vet Intern Med 34 (2020): 2056-2067.
- Tsukahara T, Kawaguchi S, Torigoe T, et al. Prognostic impact and immunogenicity of a novel osteosarcoma antigen, papillomavirus binding factor, in patients with osteosarcoma. Cancer Sci 99 (2008): 368-375.
- Li D, Toji S, Watanabe K, et al. Identification of novel human leukocyte antigen?A*11:01?restricted cytotoxic T?lymphocyte epitopes derived from osteosarcoma antigen papillomavirus binding factor. Cancer Sci 110 (2019): 1156-1168.
- Doyle HA, Gee RJ, Masters TD, et al. Vaccine-induced ErbB (EGFR/HER2)-specific immunity in spontaneous canine cancer. Transl Oncol 14 (2021): 101205.
- Xiao H, Chen L, Luo G, et al. Effect of the cytokine levels in serum on osteosarcoma. Tumor Biology 35 (2014): 1023-1028.
- Buddingh EP, Schilham MW, Ruslan SEN, et al. Chemotherapy-resistant osteosarcoma is highly susceptible to IL-15-activated allogeneic and autologous NK cells. Cancer Immunology, Immunotherapy 60 (2011): 575-586.
- Wang W, Ding H, Sun Z, et al. A population-based propensity-matched study of regional dissections in patients with metastatic osteosarcoma. J Orthop Surg Res 15 (2020): 107.
- Nasr S, McKolanis J, Pais R, et al. A phase I study of interleukin-2 in children with cancer and evaluation of clinical and immunologic status during therapy. A pediatric oncology group study. Cancer 64 (1989): 783-788.