Protective Effects of Ginsenoside Rg1 on Acute Myocardial Infarction
Article Information
Xiaoyu Wanga,b, Linlin Wangb, Lili Qia,b, Huimiao Bianb, Xing Yanc, Yan Wangc, Xiaodong Lic, Kenka Chod, Guojiang Wua,*, Baohong Jiangb,*
aCollege of Life Sciences, Hebei Agricultural University, Baoding, China
bShanghai Institute of Materia Medica, Chinese Academy of Sciences, Shanghai, China
cInstitute of Plant Physiology and Ecology, Chinese Academy of Sciences, Shanghai, China
dTakarazuka University of Medical and Health Care, Hanayashiki-Midorigaoka, Takarazuka City, Japan
*Corresponding Author(s): Guojiang Wu, College of Life Sciences, Hebei Agricultural University, 2596# Lekainan Street, Baoding, 071000, China
Baohong Jiang, Shanghai Institute of Materia Medica, Chinese Academy of Sciences, 501# Haike Road, Shanghai, 201203, China
Received: 01 June 2020; Accepted: 10 June 2020; Published: 10 August 2020
Citation:
Xiaoyu Wang, Linlin Wang, Lili Qi, Huimiao Bian, Xing Yan, Yan Wang, Xiaodong Li, Kenka Cho, Guojiang Wu, Baohong Jiang. Protective Effects of Ginsenoside Rg1 on Acute Myocardial Infarction. Journal of Pharmacy and Pharmacology Research 4 (2020): 44-57.
View / Download Pdf Share at FacebookAbstract
Context: Ginsenoside Rg1 (Rg1), as the active ingredient of Panax notoginseng, has protective effect on the cardiovascular system. While, the efficiency of Rg1 against acute myocardial infarction (MI) is not fully elucidated. The aim of this study was to investigate the cardio-protection and the underlying mechanism for Rg1.
Objective: Myocardial infarction was induced by ligation of the left anterior descending coronary artery of wistar rats. 2,3,5-triphenyltetrazolium chloride (TTC) stain assay was used to detect infarct size; hematoxylin and eosin (HE) and phosphotungstic acid-hematoxilin (PTAH) stain assay were used to evaluate the cardiac structure; terminal deoxytransferase-mediated dUTP-biotin nick end labeling (TUNEL) assay was used to detect apoptosis; immunohistochemical stain was used to evaluate neutrophils and macrophages infiltration.
Key Findings: Firstly, Extract Rg1 (P < 0.01) and Ferment Rg1 (P < 0.01) down-regulated myocardial infarct size and up-regulated superoxide dismutase (SOD) activity significantly. Then, HE and PTAH stain indicated the protection of Extract Rg1 and Ferment Rg1 on heart structure, especially on the integrity of sarcomere. Finally, both Extract Rg1 (P < 0.001) and Ferment Rg1 (P < 0.001) reduced the number of apoptosis cells, and further inhibited the infiltration of neutrophils into the infarct site of heart.
Summary: For the first time, our present study verified the efficiency of Rg1 against acute MI, and provided experimental evidence that Ferment Rg1 holds similar cardio-protection with Extract Rg1. Rg1 holds potential therapeutic value for further drug development on heart disease.
Keywords
Myocardial Infarction; Extract; Ferment; Ginsenoside Rg1
Myocardial Infarction articles, Extract articles, Ferment articles, Ginsenoside Rg1 articles
Article Details
Introduction
Myocardial infarction (MI) is that the sudden decrease or even interruption of blood flow in the coronary artery caused by coronary occlusion results in severe and persistent acute ischemia and hypoxia in the corresponding myocardium, eventually leading to ischemic myocardial necrosis. Ischemic heart disease is one of the leading causes of death at the national level in China in 2017. Around the world, there are more 20 million people dying from MI each year [1]. Currently, cardioprotective medicines against MI are deficient in clinic. We urgently need to find more candidate drugs to develop for MI patients.
Panax notoginseng, one of the most frequently used traditional Chinese medicine, is well known for its efficacy in promoting blood circulation and ameliorating pathological hemostasis [2-4]. Ginsenoside Rg1 (Rg1) is a main bioactive component of Panax notoginseng. A number of clinical and physiological effects of Rg1 have been described recently, such as preservation of myocardial structure and cardiac function by enhancing angiogenesis, and attenuating left ventricle myocardial fibrosis in the rats model of myocardial ischemia-reperfusion or MI [5-7]. Our previous animal experiment study also reported that Rg1 performed cardioprotection with inhibition of vascular remodeling, not only on large conductance artery but also on small resistance artery [8]. Previous study reported that Rg1 promoted angiogenesis and reduced the level of myocardial fibrosis in chronic cardiac injury model, but there is no report on acute protection of Rg1 against MI. Therefore, we used the rats model of acute MI to evaluate the efficacy of Rg1.
At present, there are two technical bottlenecks in obtaining Rg1 with high purity. Firstly, the repeated cultivation of Panax plants makes soil unsustainable, and can result in decreasing productivity [9]. Obstacles to replanting are prevalent among the Panax species, and replanting could fail because of high seedling death rates [10]. Soil conditions must be improved by many years of crop rotations before Panax notoginseng can be replanted, and arable soils for Panax notoginseng cultivation are becoming scarce [11]. Secondly, the similar structure of ginsenosides increases difficulty on the purification of individual component, even though Panax notoginseng is in abundant supply. In the purification process, it is difficult to obtain high purity Rg1 monomer, because of the similar structure, strong hydrophobicity of ginsenoside lead to the mutual interference in the separation and purification process [12-13]. Fermentation is a new technology which reduces the dependence on cultivation and produces high purity of Rg1, compared with the traditional extraction method. Consequently, fermentation is the most desirable method for industrial application in the condition that plant resources are scarce, and active ingredients are difficult to extract and purify. We have also been committed to using fermentation to solve the above problems on ginsenosides [9, 14].
In the present study, efficiency of Rg1 from extraction and Rg1 from fermentation were evaluated using MI rat model. It has laid a solid research foundation for Rg1 to enter the clinical and market in the future.
Methods
Materials
Healthy wistar male rats were purchased from Shanghai Center of Experimental Animals, Chinese Academy of Sciences. Pentobarbital sodium intraperitoneally was purchased from Alfasan International B.V. Extract Rg1 was purchased from Shanghai Yousi Bio-Tech Co.,Ltd. Ferment Rg1 was approved by Institute of Plant Physiology and Ecology, Chinese Academy of Sciences. TTC was purchased from Sigma Aldrich Co., Ltd. ELISA kits for SOD was purchased from Nanjing Jiancheng Bioengineering Research Institute Co., Ltd. Haematoxylin and eosin were purchased from Shanghai YiFan Blological Technology Co., Ltd. PTAH staining Kit was purchased from Beijing Solebo Technology Co., Ltd. TUNEL Apoptosis Detection Kit was purchased from Shanghai Yeasen Biotechnology Co., Ltd. CD44 antibody, CD68 antibody, Goat anti-rabbit antibody, DAB kit and DAPI were purchased from Boster Biological Technology Co., Ltd. Unless otherwise noted, solvents and reagents were purchased from Sinopharm Chemical Reagent Co., Ltd.
MI model and Rg1 treatment
32 healthy adult male wistar rats (200-230 g) were acclimatized in temperature and humidity-controlled rooms with a 12-hours dark/light cycle. Water and diet were provided adlibitum. All protocol was approved by Institutional Animal Care and Use Committee at Shanghai Institute of Materia Medica (IACUC number: 2019-03-GDA-62). After anesthetized by 40 mg/kg pentobarbital sodium intraperitoneally, rats were connected to an ALC-V9 ventilator (Alcott, Shanghai, China). The tidal volume was set as 18 mL, the respiratory rate was set as 80 breaths per minute, and the inspiration/expiration ratio was set as 1:1. MI was introduced by ligation of the left anterior descending coronary artery near the main pulmonary artery. After surgery, all MI rats were randomly divided into 3 groups: rats in MI group (MI, n=8) were given normal saline; rats in fermentation group (Ferment, n=8) were given 15 mg/kg Rg1 prepared by fermentation; rats in extraction group (Extract, n=8) were given 15 mg/kg Rg1 by extraction. Sham group (Sham, n=8) was performed using an identical procedure, except that the suture was passed under the coronary artery without ligation. Rats were injected with 15 mg/kg Rg1 intravenously once immediately after surgery, 23 hours after surgery (Supplementary Figure 1). Rats in the Sham and MI groups were given the same volume of normal saline at the same time. After intravenous administration, the rats were put on an ALC-HTP animal thermostatic system (Alcott, Shanghai, China) to maintain body temperature until they woke up.
SOD measurement
24 hours later after surgery, all rats were sacrificed by 120 mg/kg pentobarbital sodium intraperitoneally. Blood collected from the abdominal aorta was centrifuged at 8000 rpm for 10 minutes at 4°. The separated serum was used for SOD analysis using an assay kit as the recommendation of manufacture. Briefly, 20μL serum was added to 96-well plate and the diluted xanthine oxidase was separately added to each well following. After the 96-well plate was incubated for 20 minutes at 37° on a shaker, the absorbance of each well was read at 450 nm by the multimode plate reader (Perkin Elmer, Boston, USA) and expressed as International Units U/mL protein.
Left ventricle infarct size evaluation
After euthanasia, every heart was frozen at -20° for 20 minutes, then cut into 6 sections using a cold razor blade. Each section was stained with 0.5% TTC solution at 37° for 20 minutes. Morphometric measurement of infarct area was performed by image analysis system (Image-Pro Plus version 6.0.). TTC infarct size was calculated as the percentage of infarct area to the whole area heart. After TTC stain, the heart samples were fixed by 4% neutral-buffered paraformaldehyde, and then were paraffin-embedded, cut at 5 mm slices for histopathological examination.
HE staining
The tissues were dewaxed with xylene, hydrated with gradient alcohol, stained with hematoxylin for 15 minutes, differentiated with 1% alcohol hydrochloric acid for 2-3 seconds. The running water was returned to the blue for 5 minutes, and the eosin solution was immersed for 10 minutes. Afterwards, the tissues were hydrated with gradient alcohol, cleared in xylene, sealed by neutral balsam. Photomicrographs were taken using an Olympus BX51 microscope plus Olympus DP71 CCD camera (Olympus, Tokyo, Japan).
Immunohistochemical staining
Slides were deparaffinized and rehydrated, antigen retrieval was then achieved in sodium citrate buffer (pH 6.0) for 20 minutes using a microwave oven. Sections were incubated with CD44 or CD68 antibody diluted 1:100 in 1% PBS at 4° overnight following blocking of endogenous peroxidase with 3% hydrogen peroxide and preincubation with 10% normal goat serum. After removal of the primary antibodies with three 5-minutes washes in PBS, sections were incubated for 1 hour with goat anti-rabbit antibody diluted 1:200 in 1% PBS at 37 °. After three 5-minutes washes in PBS, the sections were developed with DAB kit, stopped with rinses of double-distilled water. Sections were restained with hematoxylin. Photomicrographs were taken using an Olympus BX51 microscope plus Olympus DP71 CCD camera (Olympus, Tokyo, Japan).
TUNEL apoptosis analysis
To evaluate apoptosis in infarct area of rat heart, TUNEL Apoptosis Detection Kit was used according to the manufacturer’s instructions. The sections were deparaffinized, rehydrated, and permeabilized by incubation in solutions containing proteinase K. After equilibration and labeling reaction, the ventricular specimens were immerged into the DAPI solution to stain the nuclei of cells. Laser scanning confocal microscope (Olympus, Fluoview1000, Tokyo, Japan) was used to view the fluorescence staining. Total cells were visualized at 330-380 nm for DAPI staining, and apoptotic cells were visualized at 465-495 nm for FITC staining. The ratio of positive apoptotic cells and total cells was used to express the apoptosis index.
PTAH assay
The slides were dewaxed with xylene, hydrated with gradient alcohol, oxidized with PTAH oxidizer for 5 minutes, bleached with oxalic acid solution for 90 s, and stained with Mallory PTAH staining solution for 24 hours. After 24 hours, the excess dyes were washed out with 95% ethanol, the slides were dehydrated with xylene, and sealed by neutral balsam. Photomicrographs were taken using an Olympus BX51 microscope plus Olympus DP71 CCD camera (Olympus, Tokyo, Japan).
Statistical analysis
Data analysis was performed using GraphPad Prism 5.0 (GraphPad software, LA Jolla, CA, USA). All data were expressed as mean ± standard error of mean, and one-way ANOVA was used to confirm whether the variance was homogeneous. If n were equal, the Tukey method was used for data comparison; if n were not equal, the Bonferroni method was used for data comparison, P<0.05 was statistically significant.
Results
Infarct size is an important index to evaluate myocardial damage and TTC stain is one of the standard methods to evaluate infarct size in animal models. The representative images of TTC stain were shown in Figure 1A, red regions represented non-infarct tissue, and pale white regions indicated infarct tissue. The quantification of TTC stain was shown in Figure 1B. Compared with Sham group, myocardial infarct size was increased in MI group (39.1% ± 8.0% vs. 0.0 ± 0.0%, P< 0.001) significantly. Both Extract Rg1 (25.6 ± 3.4% vs. 39.1% ± 8.0%, P < 0.01) and Ferment Rg1 (24.9 ± 9.0% vs. 39.1% ± 8.0%, P < 0.01) were reduced myocardial infarct size significantly. Heart index is one of the methods to evaluated myocardial edema. As shown in Figure 1C, compared with Sham group, the heart index showed an increasing trend in MI group, while Extract Rg1 and Ferment Rg1 did not show effects on heart index. SOD activity is another bio-marker that reflects the degree of ischemic injury. Figure 1D showed that SOD activity was reduced in MI group (119.9±11.7 U/mL vs. 164.5±14.9 U/mL, P < 0.001) compared with Sham group significantly. Both Extract Rg1 (166.4 ± 16.2 U/mL vs. 119.9±11.7 U/mL, P < 0.001) and Ferment Rg1 (151.9±12.6 U/mL vs. 119.9±11.7 U/mL, P < 0.01) up-regulated SOD activity significantly, compared with MI group.

Figure 1: Ferment Rg1 decreased infarct size and up-regulated SOD activity. A. The representative images of TTC stain. B. The quantification of TTC stain. C. Heart index of each group. D. The activity of SOD from serum was determined by ELISA. Data are presented as mean ± SE. ***p<0.001 compared with Sham group; ##p<0.01, ###p<0.001 compared with MI group. n=5-8.
After we confirmed the protective effect of both Ferment Rg1 and Extract Rg1 against myocardial infarction based on TTC stain, we further evaluated the effects of Rg1 on myocardial structure in infarct, border and remote area, respectively. Representative photomicrographs of HE stain were shown in Figure 2. In MI group, the myocardial structure was destroyed seriously at the infarct area, with the characteristics of inflammatory cells infiltration, cellular degeneration, coagulation necrosis, interstitial edema, nuclei loss. Compared with MI group, Extract Rg1 and Ferment Rg1 improved the above-mentioned damages. In the border area of MI group, inflammatory cells infiltration was also obvious, and this phenomenon had been improved by Extract Rg1 and Ferment Rg1. Cardiomyocytes aligned in an orderly arrangement without obvious difference in remote area for each group.

Figure 2: Ferment Rg1 protected the structure detected by HE stain. Representative photomicrographs of HE stain. n=7-8 for each group. Scale bar: 100 µm.
Each sarcomere is formed from a miniature, precised ordered array of parallel and partly overlapping thin and thick filaments. After we confirmed the protective effect of both Extract Rg1 and Ferment Rg1 on myocardial structure through HE stain, we further evaluated the effects of Rg1 on sarcomere with PTAH stain in infarct, border and remote area (Figure 3). In MI group, cardiac muscle cells in shortened and widened shape not only lost co-directional arrangement, but also the light bands and dark bands of sarcomere were ruptured seriously at the infarct area. In the border area of MI group, the sarcomere also showed a certain extent rupture. Both Extract Rg1 and Ferment Rg1 protected the structure of sarcomere; maintain the cardiac muscle cells in striated arrangement at the infarct and border area. There was no difference on sarcomere structure in remote area for each group.

Figure 3: Ferment Rg1 protected the sarcomere detected by PTAH stain. Representative photomicrographs of PTAH stain. n=6-8. Scale bar: 100 µm.
HE stain and PTAH stain indicated the cardio-protection of Extract Rg1 and Ferment Rg1, and then TUNEL analysis was conducted to elucidate the underlying mechanism. Representative photomicrographs of TUNEL stain were shown in Figure 4A, and TUNEL-positive cells stain green. In Figure 4B, the percentages of TUNEL-positive cells in MI group was higher than Sham group (43.9±10.5% vs. 0.0%±0.0, P<0.001). Both Extract Rg1 (16.0±2.7% vs. 43.9±10.5%, P < 0.001) and Ferment Rg1 (15.7±4.7% vs. 43.9±10.5%, P < 0.001) down-regulated the percentages of TUNEL-positive cells compared with MI group significantly.

Figure 4: Ferment Rg1 inhibited cardiomyocyte apoptosis. A. Representative photomicrographs of TUNEL stain. B. The quantification of TUNEL stain. Data are presented as mean ± SE. **p<0.01, ***p<0.001 compared with Sham group; ##p<0.01, ###p<0.001 compared with MI group. n=7-8. Scale bar: 50 μm.
Because inflammatory infiltration was observed by HE stain, we want to further detect which type of inflammatory cells involved. Representative photomicrographs of immune histochemical stain of CD44 positive cells (neutrophils, Figure 5A) and CD68 positive cells (macrophages, Figure 5B) were shown. In MI group, abundant CD44 positive cells infiltrated into infarct area, while the infiltration of CD68 positive cells was not so obvious. At the border area of MI group, sporadic distribution of CD44 positive cells was observed. Both Extract Rg1 and Ferment Rg1 inhibited neutrophils infiltration in the infarct and border area.
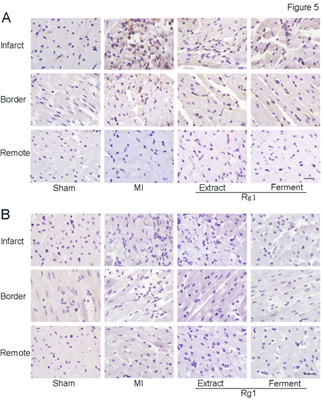
Figure 5: Ferment Rg1 inhibited the neutrophils infiltration. A. Representative photomicrographs of neutrophils immunohistochemistry stain. B. Representative photomicrographs of macrophage immunohistochemistry stain. n=6-8. Scale bar: 50 µm.
Discussion
In the present study, we found that both Extract Rg1 and Ferment Rg1 reduced myocardial infarct size, up-regulated SOD activity, protected the integrity of sarcomere, inhibited cardiomyocyte apoptosis, and attenuated neutrophils infiltration, indicating Rg1 has cardio-protection effect.
MI is a leading cause of morbidity and mortality throughout the world. At present stage, safe and effective drugs for MI are scarce in clinical. Even previous study reported that Rg1 promoted angiogenesis and reduced the level of myocardial fibrosis in chronic cardiac injury model [6-7], but there is no report on acute protection of Rg1 against MI. For the first time, we reported the efficiency of Rg1 in reducing infarct size, neutrophils infiltration on acute MI rats, which is consist with the chronic protection on myocardial fibrosis.
Neutrophils always are the first kind of leukocytes to be recruited into sites of MI injury [15]. These neutrophils, whilst vital for host defense against pathogens, are implicated in the pathogenesis of many disorders due to their capacity to release a wide range of proinflammatory mediators and reactive oxygen species [16-18]. After MI, the body usually initiates the inflammatory immune response and subsequent injury repair process, and eventually replacing the necrotic myocardium by cicatrices [19]. Our results showed the evidence that both Ferment Rg1 and Extract Rg1 inhibited the infiltration of neutrophils, indicating the further protection against cardiac remodeling. Oxygen free radical explosion is one of the main mechanisms of MI [20]. SOD is a specific enzyme for scavenging oxygen free radicals in vivo, and SOD activity indirectly reflect the ability of the body to scavenge oxygen free radicals. A decrease in SOD activity results in the decreased removal of superoxide anions, which is harmful to the myocardium [21].
Previous report showed that the myocardial protection of Rg1 during cardiomyocytes hypoxia/reoxygenation was partially due to its anti-oxidative effect [22]. Our results further confirmed that Ferment Rg1 and Extract Rg1 up-regulated SOD activity and protected myocardial cells from oxygen free radical damage. In addition, SOD also mediates cardiomyocyte apoptosis. Myocardial cell apoptosis is the main form of cell loss in the early stage [23]. Because of the persistent apoptosis, a large number of cardiac myocytes are lost and the cardiac function will be destroyed [24-25]. Rupture of the sarcomere is another indicator of dysfunction of heart. Ferment Rg1 and Extract Rg1 not only inhibited apoptosis of myocytes, but also kept the integrity of sarcomere, implying the protection of Rg1 on heart contractility. In the future study, we will evaluate the efficiency of Rg1 on heart function.
Conclusions
In summary, both Extract Rg1 and Ferment Rg1 improved cardiac structure and inhibited neutrophils infiltration significantly in rats with MI injury. Ferment Rg1 holds similar efficiency with Extract Rg1 on cardio-protection, indicating the unequivocal biological activity and clear mechanism support Rg1 for future clinical development.
Abbreviations
- MI: Myocardial infarction
- Rg1: Ginsenoside Rg1
- TTC: 2,3,5-Triphenyltetrazolium chloride
- SOD: Superoxide dismutase
- H&E: Haematoxylin and eosin
- PTAH: Phosphotungstic acid-hematoxylin
- TUNEL: Terminal deoxytransferase-mediated dUTP-biotin nick end labeling
- FITC: Fluorochromes fluorescein isothiocyanate
Declarations
Conflict of interest
The Authors declare that there is no conflict of interest.
Funding
This work was supported by National Natural Science Foundation of China (81770478, 81573646), Shanghai Science and Technology Development Foundation (16401901800), Biological Resources Programme, Chinese Academy of Sciences (KFJ-BRP-008). All these funding bodies provided necessary financial support in the design of the study and collection, analysis and interpretation of data.
Acknowledgements
We would like to thank Pro. Xing Yan for his technical assistance in fermentation. We also like to thank all the members of Pharmacological Laboratory for their valuable discussions.
Consent to publish
Not Applicable.
Availability of data and materials
The datasets used and/or analyzed during the current study are available from the corresponding author on reasonable request. All materials used in this study are properly included in Methods section.
References
- Sahoo S, Losordo DW. Exosomes and cardiac repair after myocardial infarction. Circulation Research 114 (2014): 333-344.
- Dong TT, Cui XM, Song ZH, et al. Chemical assessment of roots of Panax notoginseng in China: regional and seasonal variations in its active constituents. Journal of Agricultural and Food Chemistry 51 (2003): 4617-4623.
- Ng TB. Pharmacological activity of sanchi ginseng (Panax notoginseng). Journal of Pharmacy and Pharmacology 58 (2006): 1007-1019.
- Gillis CN. Panax ginseng pharmacology: a nitric oxide link?. Biochemical Pharmacology 54 (1997): 1-8.
- Li L, Pan CS, Yan L, et al. Ginsenoside Rg1 ameliorates rat myocardial ischemia-reperfusion injury by modulating energy metabolism pathways. Frontiers in Physiology 9 (2018): 78.
- Wei HJ, Yang HH, Chen CH, et al. Gelatin microspheres encapsulated with a nonpeptide angiogenic agent, ginsenoside Rg1, for intramyocardial injection in a rat model with infarcted myocardium. Journal of Controlled Release 120 (2007): 27-34.
- Wang XD, Gu TX, Shi EY, et al. Effect and mechanism of panaxoside Rg1 on neovascularization in myocardial infarction rats. Chinese Journal of Integrative Medicine 16 (2010): 162-166.
- Chen H, Yin J, Deng Y, et al. The protective effects of ginsenoside Rg1 against hypertension target-organ damage in spontaneously hypertensive rats. BMC Complementary and Alternative Medicine 12 (2012): 53.
- Wang P, Wei W, Ye W, et al. Synthesizing ginsenoside Rh2 in Saccharomyces cerevisiae cell factory at high-efficiency. Cell Discovery 5 (2019): 1-4.
- Ying YX, Ding WL, Zhou YQ, et al. Influence of Panax ginseng continuous cropping on metabolic function of soil microbial communities. Chinese Herbal Medicines 4 (2012): 329-334.
- Dong LL, Jiang X, Feng G, et al. Soil bacterial and fungal community dynamics in relation to Panax notoginseng death rate in a continuous cropping system. Sci Rep 6: 31802.
- Zhang L, Wang S, Qu B, et al. Efficient separation determination of protopanaxatriol ginsenosides Rg1, Re, Rf, Rh1, Rg2 by HPLC. Journal of Pharmaceutical and Biomedical Analysis 170 (2019): 48-53.
- Qu B, Zhang L, Wang S, et al. Retention behavior of ginsenosides in a sulfo-based high performance liquid chromatography column. Journal of Chromatography A 1610 (2020): 460542.
- Wang P, Wei Y, Fan Y, et al. Production of bioactive ginsenosides Rh2 and Rg3 by metabolically engineered yeasts. Metabolic Engineering 29 (2015): 97-105.
- Vermeren S, Karmakar U, Rossi AG. Immune complex-induced neutrophil functions: A focus on cell death. European Journal of Clinical Investigation 48 (2018): e12948.
- Tecchio C, Cassatella MA. Neutrophil-derived chemokines on the road to immunity. InSeminars in Immunology 28 (2016): 119-128.
- Tecchio C, Micheletti A, Cassatella MA. Neutrophil-derived cytokines: facts beyond expression. Frontiers in immunology 5 (2014): 508.
- Winterbourn CC, Kettle AJ, Hampton MB. Reactive oxygen species and neutrophil function. Annual Review of Biochemistry 85 (2016): 765-792.
- Frangogiannis NG. The mechanistic basis of infarct healing. Antioxidants & redox signaling 8 (2006): 1907-1939.
- Asaikumar L, Vennila L, Akila P, et al. Preventive effect of nerolidol on isoproterenol induced myocardial damage in Wistar rats: Evidences from biochemical and histopathological studies. Drug Development Research 80 (2019): 814-823.
- Sharma N, Rutherford JD, Grayston JT, et al. Association between C-reactive protein, anti-Chlamydia pneumoniae antibodies, and vascular function in healthy adults. American Journal of Cardiology 87 (2001): 119-121.
- Zhu D, Wu L, Li CR, et al. Ginsenoside Rg1 protects rat cardiomyocyte from hypoxia/reoxygenation oxidative injury via antioxidant and intracellular calcium homeostasis. Journal of Cellular Biochemistry 108 (2009): 117-124.
- Kajstura J, Cheng W, Reiss K, et al. Apoptotic and necrotic myocyte cell deaths are independent contributing variables of infarct size in rats. Laboratory investigation; a Journal of Technical Methods and Pathology 74 (1996): 86-107.
- Lim H, Fallavollita JA, Hard R, et al. Profound apoptosis-mediated regional myocyte loss and compensatory hypertrophy in pigs with hibernating myocardium. Circulation 100 (1999): 2380-2386.
- Abbate A, Biondi-Zoccai GG, Bussani R, et al. Increased myocardial apoptosis in patients with unfavorable left ventricular remodeling and early symptomatic post-infarction heart failure. Journal of the American College of Cardiology 41 (2003): 753-760.
Description of Data
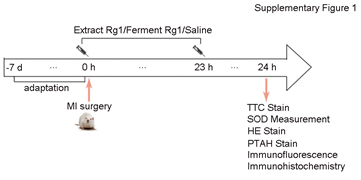
Figure 1S: After the rats adapted to environment for 7 days, we carried out the experimental operation according to the above experimental protocol. Sham group was performed using an identical procedure, except that the suture was passed under the coronary artery without ligation.

Figure 2S: The HPLC analysis was performed on a Shimadzu LC20A system (Shimadzu, Kyoto, Japan) equipped with a LC20ADXR pumper, an auto-sampler and a diode array detector. Chromatographic separation of Rg1 was carried out at 35° on a Shim-pack XR-ODS column (100 mm×2.0 mm, 2.2 μm, Shimadzu, Kyoto, Japan). The gradient elution system consisted of water (A) and acetonitrile (B). Separation was achieved using the following gradient: 0-2 min (15% B), 2-16 min (15%-70% B), 16-20 min (95% B), 20-22 min (15% B) and the flow rate were kept at 0.45 mL/min. The purity of Rg1 prepared by both methods was more than 98%.
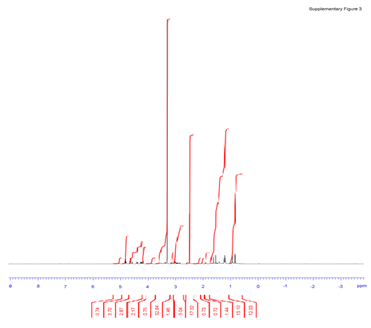
Figure 3S: In brief, 1H NMR was recorded on Bruker Avance III 500 MHz in CD3OD, and the chemical shift (δ) of 1H NMR was given in ppm relative to CD3OD (δ = 3.31 ppm). 1H NMR (500 MHz, CD3OD) δ ppm: 5.11 (t, J = 6.9 Hz, 1H), 4.61(d, J = 7.8 Hz, 1H), 4.35 (d, J = 7.8 Hz, 1H), 4.10 (td, J1 = 10.5 Hz, J2 = 3.0 Hz, 1H), 3.83-3.76 (m, 2H), 3.70-3.62 (m, 3H), 3.38-3.24 (m, 6H), 3.22-3.18 (m, 2H), 3.12-3.06 (m, 2H), 1.96-1.57 (m, 15H), 1.51-1.48 (m, 1H), 1.42-1.38 (m, 1H), 1.35 (s, 3H), 1.33 (s, 3H), 1.22-1.04 (m, 7H), 1.01 (s, 3H), 1.00 (s, 3H), 0.96 (s, 3H).