Metadichol® A Nano Lipid Emulsion that Expresses All 49 Nuclear Receptors in Stem and Somatic Cells
Article Information
Palayakotai R. Raghavan1*
1Nanorx Inc, PO Box 1131, Chappaqua, NY 10514, USA
*Corresponding author: Palayakotai R. Raghavan. Nanorx Inc, PO Box 1131, Chappaqua, NY 10514, USA.
Received: 03 October 2023; Accepted: 10 October 2023; Published: 25 October 2023.
Citation: Palayakotai R. Raghavan. Metadichol® A Nano Lipid Emulsion that Expresses All 49 Nuclear Receptors in Stem and Somatic Cells. Archives of Clinical and Biomedical Research. 7 (2023): 543-555.
View / Download Pdf Share at FacebookAbstract
Human nuclear receptors (NRs) involve 49 ligand-dependent transcription factors that are important for regulating the cell cycle and processes. There are many literature references on NR expression in many organs, abnormal cells, and tissues. However, a simple universal method to study the expression of NR is still missing. Here, we present systematic profiling of NRs in human umbilical cord stem cell lines and assess the expression of the 49 human NRs by quantitative real-time (qRT)-PCR using Metadichol, a nanoemulsion made of natural lipid alcohol. Metadicholtreated umbilical cord cells and fibroblasts, where all cells expressed NRs at a concentration range of 1 picogram per ml to 100 ng per ml in a dose dependent manner, were detected by qRT-PCR and qualified by Western blotting. This method will allow the study of many organs and tissues and expand our understanding of the role of NRs in mitigating diseases.
Highlights: Metadichol treatment of somatic cells leads to all 49 nuclear receptors being expressed.
Depending on the cell and concentration of Metadichol used, fold changes are different.
The method could be used to study many cells and disease cells to better understand NR expression patterns and implications.The results suggest that Metadichol is a universal ligand to all nuclear receptors.
Metadichol is controlling regulation of the expression of Nuclear receptors.
Keywords
Nuclear receptors; Stem cells; Fibroblasts; Ahr; Vitamin C; Sertolli cells; Chromatin; Qrt-Pcr; Metadichol; Nanoemulsion
Nuclear receptors articles; Stem cells articles; Fibroblasts articles; Ahr articles; Vitamin C articles; Sertolli cells articles; Chromatin articles; Qrt-Pcr articles; Metadichol articles; Nanoemulsion articles
Article Details
1. Introduction
Nuclear receptors (NRs) are transcription factors usually activated by small lipophilic molecules [1]. The 49 AHR (aryl hydrocarbon receptor) NRs, which are highly conserved in the human genome, are subdivided into seven subfamilies based on amino acid sequences (NOR, NR1, NR2, NR3, NR4, NR5, and NR6) [2]. Many NRs are classified as orphan receptors because they lack a specific ligand for activation [3]. Unliganded NRs are bound to heat shock protein 90 (HSP90) and can be found in the cytosol and nucleus. Receptors may also bind to corepressors without ligands [4]. 1,25-dihydroxyvitamin D3 is a small molecule that can diffuse through the cell membrane and bind to NRs located in the cytosol or nucleus of the cell [5]. This binding leads to several downstream events that eventually result in up- or downregulation of gene expression. The NR ligands exhibit a broad spectrum of full, partial, inverse agonist, or antagonist activities. Selective NR modulators activate only a subset of the functions induced by the ligand or act in a cell-type-selective manner [6]. Chromatin plays a crucial role in the actions of NR by modulating interactions with regulatory elements in the genome. However, when receptor binding occurs, chromatin changes that impact receptor signaling [7]. NRs bind to sDNA sequences, leading to modification of the chromatin structure. The final outcome leads to modulation of RNA polymerase activity resulting in increased or decreased transcription. transcription (Zaret and Yamamoto, 1984). Chromatin modification leads to pathways that impact NR action in various cells. Chromatin remodeling leads to numerous processes that involve pluripotency, cellular differentiation, inflammation, DNA damage and repair and tumor suppression [8]. NR regulation and its tissue-expression profile along with the associated cofactors are necessary to separate desirable therapeutic efficacies from undesirable side effects. One of the least studied areas is the regulation of NR ligands by NRs themselves. [8] reported NR expression profiles in human and mouse embryonic stem cell lines and during their early differentiation into embryoid bodies [9]. The expression of the 49 human and mouse NRs was assessed by quantitative real-time (qRT)-PCR. Hong et al. evaluated the expression of the receptors for estrogen, progesterone, and glucocorticoids [10]. Very little is known about systemic NR expression in human cell lines. In this research, we present the specific roles of NRs in human cell lines by characterizing the RNA and cDNA expression profiles of the NR superfamily while treating stem cells and fibroblasts with metadichol [11], which is a nanoemulsion of long-chain alcohols [12], using qRT-PCR and Western blotting methods.
2. Methods:
The methods, including qRT-PCR, Western blotting, and cell culture, were carried out by a commercial service provider, Skanda Life sciences, Bangalore, India. The procurement of chemicals and reagents was as follows. Human mesenchymal stem cells and normal human dermal fibroblast cells were procured from ATCC (USA). Primary antibodies were from either ABclonal, Woburn, (both in Massachusetts, USA) or Elabscience, Maryland, USA. The primers were from Sahagene, Hyderabad, India. Other molecular biology reagents were from Sigma-Aldrich, India.
2.1 Cell maintenance and seeding:
We preserved the cells in a suitable medium with 1% antibiotics in a wet atmosphere of 5% CO2 at 37 °C. We changed the medium every two days until the cells reached confluency, and we assessed their viability using a hemocytometer. At 70%-80% confluency, we prepared and seeded single-cell suspensions containing 106 cells/mL in six-well plates at a density of 106 cells/well. We incubated the cells for 24 h at 37 °C in 5% CO2, rinsed the cell monolayer with serum-free medium and treated it with Metadichol at preset concentrations.
2.2 Cell treatment:
We prepared Metadichol in serum-free media at different concentrations (1 pg/mL, 100 pg/mL, 1 ng/mL, and 100 ng/mL) and then added them to predesignated wells. Note that the control cells were drug-free. We incubated the cells for 24 h. Next, we washed them gently with sterile PBS. We isolated RNA using TRIzol following the manufacturer’s instructions and prepared cDNA followed by the analysis of several biomarkers using qPCR and Western blotting.
2.2 RNA isolation:
We separated total RNA using TRIzol reagent (Invitrogen) and collected approximately 106 cells in 1.5 mL microcentrifuge tubes. The cells were centrifuged at 5,000 rpm for 5 min at 4 °C, and the supernatant was removed. Next, we added 650 µL of TRIzol to the pellet and mixed the solution to be incubated on ice for 20 min. Subsequently, we added 300 µL of chloroform and mixed the samples well by gentle inversion for 1–2 min and then incubated them on ice for 10 min. We centrifuged the samples at 12,000 rpm for 15 min at 4 °C and carefully transferred the upper aqueous layer to a new sterile 1.5 mL centrifuge tube, to which we added an equal amount of prechilled isopropanol and incubated the samples at −20 °C for 60 min. Afterward, we centrifuged the mixture at 12,000 rpm for 15 min at 4 °C and prudently removed the supernatant. The RNA pellets were retained and washed with 1.0 mL of 100% ethanol, followed by 700 µL of 70% ethanol via centrifugation as described previously after each step. We air-dried the RNA pellets at room temperature for almost 15–20 min and then resuspended them in 30 µL of DEPC-treated water. We quantified the RNA concentration using a SpectraMax i3x . SpectraDrop Micro-Volume Microplate (Molecular Devices, USA) and synthesized cDNA using reverse transcription-PCR.
2.3 cDNA synthesis:
Following the manufacturer’s guidelines, we synthesized cDNA from 2 µg of RNA using the PrimeScript cDNA synthesis kit (Takara, France) and oligo dT primers. The reaction volume was 20 μL, and cDNA synthesis was performed at 50 °C for 30 min, followed by 85 °C for 5 min on an Applied Biosystems instrument (Veritii). The obtained cDNA was used in the following step for qPCR.
2.4 Primers and qPCR:
The PCR blend (final volume of 20 µL) contained 1 µL of cDNA, 10 µL of SYBR green Master Mix, and 1 µM complementary primers specific for the particular target genes. We ran the samples at the following settings: primary denaturation at 95 °C for 5 min followed by 30 cycles of a secondary at 95 °C for 30 s, then hardening at the optimized temperature for 30 s, with an extension at 72 °C for 1 min. We identified the number of cycles that allowed amplification in the exponential range without reaching a plateau as the optimal number of cycles. We evaluated the results using CFX Maestro software and computed the fold change via the following equation.
2.5 ΔΔCT method:
We determined the relative expression of the target gene in relation to the housekeeping gene (β-actin) and untreated control cells by the comparative CT method.
The ΔCT for each treatment was calculated using the formula:
ΔCT = CT (target gene) – CT (reference genes).
To obtain a ΔΔCT, we subtracted the individual samples between the treated and control groups as follows:
ΔΔCT = ΔCT (treatment group) – ΔCT (control group).
Similarly, we calculated the fold change in the target gene expression for each treatment using the formula.
Fold change = 2^ (-ΔΔCT)
2.6 Protein isolation:
We isolated total protein from 106 cells using RIPA buffer supplemented with the protease inhibitor PMSF. We applied a mild inversion for 30 min at 4 °C to lyse the cells and then centrifuged them at 10,000 rpm for 15 min. Finally, we transferred the supernatant to a fresh tube and determined the protein concentration using the Bradford method, where 25 µg of protein was mixed with 1X sample loading dye containing SDS and loaded on a gel. Under denaturing conditions, we separated the proteins using Tris-glycine running buffer.
2.7 Western blotting:
We transferred the proteins to methanol activated PVDF membranes (Invitrogen, USA) using a Turbo transblot system (Bio Rad, USA). We blocked the membranes with 5% BSA for 1 h and incubated them with the respective primary antibody overnight at 4 °C followed by a species-specific secondary antibody for 1 h at room temperature. We rinsed the blots and incubated them with ECL substrate (Merck, USA) for 1 min in the dark and captured the images at suitable exposure settings using a ChemiDoc XRS system (Bio Rad, USA).
Table 1: List of primers used for Q-RT-PCR
Gene |
Primers |
Base pairs |
|
NR1C2/PPARD |
F |
CCTTCTCAAGTATGGCGTGC |
226 |
R |
GATGGCCGCAATGAATAGGG |
||
RXRG |
F |
CAGGAAAGCACTACGGGGTA |
254 |
R |
CCTCACTCTCAGCTCGCTCT |
||
PPARG |
F |
AGAAGCCTGCATTTCTGCAT |
236 |
R |
TCAAAGGAGTGGGAGTGGTC |
||
NR2F1 |
F |
CATTTTTGGGCGATCTCCAGG |
261 |
R |
GCCTTCTTCTTTCGGGAGGT |
||
HNF4A |
F |
ACTGCCACGTACCTGTGCCT |
274 |
R |
AGGCATGCGAGTTGTGACCA |
||
HNF4G |
F |
AGCTGGCATATCTCAGCTGGC |
185 |
R |
AACACCTGGCTGGCAATCGG |
||
NR2F2 |
F |
CTCAACTGCCACTCGTACCT |
253 |
R |
TCAACACAAACAGCTCGCTC |
||
NR1I3 |
F |
CAGCAAACACCTGTGCAACT |
189 |
R |
TGCGAAGTGTGTGACCAGAG |
||
NR1H4 |
F |
AACAGAACAAGTGGCAGGTC |
201 |
R |
AGAGTCTCAGCTGGCATACG |
||
ESR1 |
F |
GATGTGGGAGAGGATGAGGA |
165 |
R |
TCAGGCATGCGAGTAACAAG |
||
ESR2 |
F |
TTCAGCCTGTGACCTCTGTG |
178 |
R |
CTTGGTTTGTCCAGGACGTT |
||
ESRRA |
F |
CAGGGGAGCATCGAGTACAG |
303 |
R |
CTTCTCAGGCTCAACCACCA |
||
NR1D2 |
F |
AGTTCTTCCAGCTCAGCCTC |
226 |
R |
TTGTCATCCCAGGTGCACTC |
||
ESRRB |
F |
CTTGGTTTGTCCAGGACGTT |
264 |
R |
TTTTCCATCATGGCTTGACA |
||
NR5A2 |
F |
TCCAGCTTCCAGGCAGCCTC |
234 |
R |
GATCTGTGAATCTGCGTT |
||
NR3C2 |
F |
CTGCCTCGTTTCCCTTTTCC |
231 |
R |
CCATGATCTGTGCGTTCCTG |
||
NR0B2 |
F |
GCTGTCTGGAGTCCTTCTGG |
164 |
R |
CTGGGTATGAATCCCAGCAC |
||
ESRRG |
F |
GACTTGACTCGCCACCTCTC |
174 |
R |
GTGGTACCCAGAAGCGATGT |
||
NR4A2 |
F |
CCGGTGTCTAGTTGCCAGAT |
275 |
R |
ACGCCGTAGTGTTGGCAG |
||
NR2F6 |
F |
GGACTCTGGCTTCTCTCCTC |
187 |
R |
TAGGGGTGCTGAGGAACAAG |
||
NR6A1 |
F |
GAGGAACAGGTGCCAGTACT |
175 |
R |
GGCCTCTTCCTCAAACTCCT |
||
THRB |
F |
GCCTCCAATAGCTCCAGGAT |
201 |
R |
CACCCAGTTCCAGGATTCCT |
||
VDR |
F |
GACGCCCACCATAAGACCTA |
247 |
R |
AGATTGGAGAAGCTGGACGA |
||
NR1H2 |
F |
CCTCCTGAAGGCATCCACTA |
261 |
R |
GAACTCGAAGATGGGGTTGA |
||
NR1D1 |
F |
AGGCAGCAAGCAAGCAGT |
291 |
R |
ACAGCGCATCCTTCCCCATA |
||
NR2C1 |
F |
CCCAAGGCAAGCAGTTCATT |
157 |
R |
GCAGACAGATCAGGAGTGGT |
||
NR2C2 |
F |
TCACCACCTCAGACAACCTC |
164 |
R |
ACTGACAGCCCCATAGTGAC |
||
NR1I2 |
F |
AACGCAGATGAGGAAGTCGG |
103 |
R |
AGCCCTTGCATCCTTCACAT |
||
NR4A3 |
F |
GCCCAATATAGCCCTTCCCC |
224 |
R |
TGCATTTGGTACACGCAGGA |
||
NR3C1 |
F |
CTTGCATATTTGTGCCTTCA |
174 |
R |
CTTGATGATTTGTGTTGTGC |
||
AR |
F |
GGGGCTAGACTGCTCAACTG |
169 |
R |
GCCAAGTTTTGGCTGAAGAG |
||
NR0B1 |
F |
CAGAGGCCAGGGGGTAAAG |
137 |
R |
TGCGCTTGATTTGTGCTCGT |
||
PGR |
F |
ACATGGTAGCTGTGGGAAGG |
198 |
R |
GCTAAGCCAGCAAGAAATGG |
||
RORA |
F |
TCGAACCAGTAGAAACCGCT |
219 |
R |
TTGGCCGAGATGTTGTAGGT |
||
RORB |
F |
CTCACTTCTCCACCTGCTCA |
212 |
R |
GGAGTTGGTGGCTGGGATAT |
||
RORC |
F |
AGTCGGAAGGCAAGATCAGA |
204 |
R |
CAAGAGAGGTTCTGGGCAAG |
||
NR2E3 |
F |
GGAGTCCAACACTGAGTCCC |
289 |
R |
GGCCATGAAGAGTAGGCGAG |
||
NR5A1 |
F |
AGGCACCAGGGAAGATCA |
241 |
R |
TGCCAGGCCAGGGAATACA |
||
NR2E1 |
F |
CAAGTGGGCTAAGAGTGTGC |
158 |
R |
CGTTCATGCCAGATACAGCC |
||
NR4A1 |
F |
GCCAATCTCCTCACTTCCCT |
202 |
R |
CAGCAAAGCCAGGGATCTTC |
||
RARA |
F |
GTGTCACCGGGACAAGAACT |
146 |
R |
CGTCAGCGTGTAGCTCTCAG |
||
RXRA |
F |
CTCTGTTGTGTCCTGTTGCC |
155 |
R |
CTTCTCCCTTTGCGTGTTCC |
||
PPARA |
F |
CTGTCTGCTCTGTGGACTCA |
247 |
R |
AGAACTATCCTCGCCGATGG |
||
RARB |
F |
GGTTTCACTGGCTTGACCAT |
216 |
R |
GGCAAAGGTGAACACAAGGT |
||
AHR |
F |
GGTTTCACTGGCTTGACCAT |
274 |
R |
CAGAGGACCAAATCCAGCAT |
||
RARG |
F |
GAAGACCGCGACACAACTTCC |
180 |
R |
GTTGAGTTAAGACATGAGGG |
||
RXRB |
F |
GCAGGAGTAGGAGCCATCTT |
188 |
R |
GCATACACTTTCTCCCGCAG |
||
THRA |
F |
ACCTCCATCCCACCTATTCC |
242 |
R |
CTCTTCAGGAGTGGGCTCTG |
||
NR1H3 |
F |
GAGATCCTCCCGTGGCATTA |
151 |
R |
GAGAACCCTGTGCAAAGTGG |
F, forward; R, reverse
Table 2: Metadichol and Human Mesenchymal Stem Cells and fold changes of NR’s
Metadichol concentrations |
1 pg |
100 pg |
1 ng |
100 ng |
|
Common name |
Nomenclature name |
||||
0.19 |
0.33 |
0.38 |
0.11 |
NR0B1 |
|
1.39 |
0.75 |
1.06 |
0.29 |
NR0B2 |
|
16.16 |
12.24 |
7.7 |
5.32 |
NR1A1 |
|
7.71 |
1.94 |
15.11 |
8.71 |
NR1A2 |
|
1.27 |
0.79 |
0.52 |
0.44 |
NR1B1 |
|
RARB |
1.67 |
1.39 |
0.48 |
0.73 |
NR1B2 |
2.52 |
1.04 |
0.96 |
0.82 |
NR1B3 |
|
4.66 |
3.48 |
3.92 |
1.35 |
NR1C1 |
|
3.74 |
4.5 |
4.5 |
0.44 |
NR1C2 |
|
PPARG |
1.82 |
1.7 |
1.03 |
1 |
NR1C3 |
1.93 |
1.29 |
0.8 |
0.6 |
NR1D1 |
|
0.89 |
0.47 |
0.35 |
0.15 |
NR1D2 |
|
1.77 |
1.39 |
0.94 |
0.67 |
NR1F1 |
|
0.81 |
0.84 |
0.7 |
0.33 |
NR1F2 |
|
0.52 |
0.74 |
1.19 |
1.08 |
NR1F3 |
|
LXRB |
1.28 |
0.97 |
0.55 |
0.19 |
|
1.28 |
1.17 |
0.84 |
0.18 |
NR1H3 |
|
FXR |
1.98 |
1.09 |
0.53 |
0.6 |
NR1H4 |
2.03 |
0.92 |
3.67 |
0.54 |
NR1I1 |
|
0.6 |
0.74 |
1.11 |
0.39 |
NR1I2 |
|
8.03 |
1.49 |
2.91 |
10.61 |
NR1I3 |
|
HNF4A |
0.99 |
0.72 |
0.51 |
0.13 |
NR2A1 |
1.39 |
1.51 |
0.36 |
0.26 |
NR2A2 |
|
RXRA |
1.4 |
1.21 |
0.99 |
0.79 |
NR2B1 |
RXRB |
1.87 |
1.13 |
1.05 |
0.69 |
NR2B2 |
RXRG |
2.15 |
2.2 |
1.5 |
0.76 |
NR2B3 |
1.3 |
1.27 |
0.74 |
0.39 |
NR2C1 |
|
1.6 |
1.5 |
0.74 |
0.51 |
NR2C2 |
|
0.95 |
1.37 |
1.18 |
0.57 |
NR2E1 |
|
2.18 |
1.23 |
1.51 |
1.25 |
NR2E3 |
|
1.78 |
1.57 |
1.04 |
0.65 |
NR2F1 |
|
1.81 |
1.48 |
1.15 |
1.07 |
NR2F2 |
|
0.98 |
0.95 |
0.43 |
0.08 |
NR2F6 |
|
1.86 |
1.17 |
1.94 |
0.4 |
NR3A1 |
|
1.81 |
1.37 |
1.09 |
0.66 |
NR3A2 |
|
1 |
0.88 |
0.59 |
0.35 |
NR3B1 |
|
1.11 |
2.32 |
1.45 |
1.36 |
NR3B2 |
|
1.84 |
1.02 |
0.55 |
0.18 |
NR3B3 |
|
0.99 |
0.86 |
0.82 |
0.09 |
NR3C1 |
|
1.15 |
0.78 |
0.52 |
0.21 |
NR3C2 |
|
1.19 |
0.94 |
0.67 |
0.12 |
NR3C3 |
|
1.15 |
0.37 |
0.11 |
0.33 |
NR3C4 |
|
1.82 |
0.67 |
1.16 |
0.61 |
NR4A1 |
|
1.06 |
0.61 |
0.45 |
0 |
NR4A2 |
|
5.43 |
1.89 |
0.35 |
0.5 |
NR4A3 |
|
3.2 |
2.56 |
1.92 |
5.09 |
||
LRH1 |
1.3 |
0.72 |
0.29 |
0.15 |
NR5A2 |
1.7 |
0.86 |
0.65 |
0.15 |
NR6A1 |
|
AHR |
0.39 |
0.58 |
0.79 |
0.51 |
AHR |
Table 3: Metadichol and normal human dermal fibroblasts and Fold changes of NR’s
Metadichol concentrations |
1pg |
100pg |
1ng |
100ng |
|
Common Name |
Nomenclature |
||||
1.76 |
0.14 |
0.44 |
0.24 |
NR0B1 |
|
No Detectable expression |
No Detectable expression |
No Detectable expression |
No Detectable expression |
NR0B2 |
|
1.2 |
0.65 |
0.55 |
0.69 |
NR1A1 |
|
1.15 |
1.18 |
2.4 |
0.92 |
NR1A2 |
|
2.15 |
1.81 |
0.63 |
1.06 |
NR1B1 |
|
RARB |
2.68 |
1.3 |
1.18 |
1.1 |
NR1B2 |
2.84 |
2.95 |
3.9 |
1.09 |
NR1B3 |
|
1.9 |
1.24 |
0.72 |
1.8 |
NR1C1 |
|
2.48 |
2.59 |
2.83 |
0.84 |
NR1C2 |
|
PPARG |
3.78 |
6.11 |
7.31 |
3.07 |
NR1C3 |
1.5 |
0.64 |
0.07 |
0.75 |
NR1D1 |
|
2.19 |
1.18 |
1.8 |
1.82 |
NR1D2 |
|
0.9 |
1.14 |
1.33 |
1.01 |
NR1F1 |
|
2.71 |
0.99 |
0.69 |
0.88 |
NR1F2 |
|
1.86 |
1.02 |
0.91 |
1.04 |
NR1F3 |
|
LXRB |
4.95 |
1.03 |
0.03 |
1.71 |
|
1.63 |
1.79 |
3.84 |
0.71 |
NR1H3 |
|
FXR |
2.69 |
1.32 |
1.27 |
0.87 |
NR1H4 |
1.83 |
2.34 |
2.35 |
1.54 |
NR1I1 |
|
1.81 |
0.37 |
0.97 |
1 |
NR1I2 |
|
0.98 |
0.67 |
0.49 |
0.53 |
NR1I3 |
|
HNF4A |
6.03 |
3.4 |
2.69 |
3.64 |
NR2A1 |
2.15 |
1.39 |
1.2 |
1.95 |
NR2A2 |
|
RXRA |
2.5 |
0.86 |
1.32 |
0.98 |
NR2B1 |
RXRB |
4.21 |
1.65 |
1.03 |
2.7 |
NR2B2 |
RXRG |
2.84 |
2.95 |
3.9 |
1.09 |
NR2B3 |
1.08 |
1.16 |
1.7 |
0.85 |
NR2C1 |
|
3.66 |
1.38 |
1.09 |
4.17 |
NR2C2 |
|
3.38 |
1.49 |
1.69 |
0.88 |
NR2E1 |
|
1.43 |
1.46 |
2.48 |
1.27 |
NR2E3 |
|
0.16 |
0.9 |
4.18 |
0.5 |
NR2F1 |
|
1.05 |
1.19 |
2.98 |
0.59 |
NR2F2 |
|
2.84 |
1.22 |
1.66 |
1.02 |
NR2F6 |
|
2.42 |
1.58 |
0.73 |
2.02 |
NR3A1 |
|
3.04 |
3.04 |
1.25 |
1.52 |
NR3A2 |
|
10.31 |
8.3 |
6.58 |
11.1 |
NR3B1 |
|
0.92 |
0.74 |
0.48 |
1.76 |
NR3B2 |
|
0.81 |
1.07 |
1.86 |
0.45 |
NR3B3 |
|
1.71 |
0.14 |
0.77 |
0.45 |
NR3C1 |
|
3.7 |
0.26 |
1.04 |
0.42 |
NR3C2 |
|
0.71 |
1.25 |
2.19 |
0.51 |
NR3C3 |
|
2.54 |
0.15 |
0.57 |
0.11 |
NR3C4 |
|
2.36 |
1.55 |
0.93 |
3.42 |
NR4A1 |
|
3.56 |
6.02 |
8.63 |
7.62 |
NR4A2 |
|
2.14 |
0.72 |
1.48 |
1.59 |
NR4A3 |
|
27.27 |
13.78 |
13.28 |
2.69 |
||
LRH1 |
3.32 |
1.79 |
1.74 |
2.02 |
NR5A2 |
2.46 |
0.83 |
0.39 |
2.19 |
NR6A1 |
|
AHR |
10.17 |
4.32 |
3.79 |
1.52 |
AHR |
Discussion:
Assuming a threshold of plus or minus 50% for the fold increase/decrease, 25 NRs were upregulated with stem cells and 36 with fibroblasts at a one pg ( picogram per ml) concentration. SF1 (NR5A1) showed the highest increase of 27-fold in fibroblasts and 5-fold in stem cells. NR5A1 plays an essential role in Steroidogenesis, a biological process by which the body needs key steroid hormones for functioning that are generated from cholesterol. This process affects sexual differentiation, reproduction, fertility, hypertension, obesity, and physiological homeostasis [13]. NR5A1 guides somatic cells to fetal Sertoli cells [14]. The latter are vital nurse cells located in the testis that aim at controlling spermatogenesis, i.e., production of the male germ cells. Sertoli cells also secrete cytokines and growth factors; therefore, they control immune processes that defend germ cells from immunological attack [15,16,17]. Additionally, they aid in blocking the multiplication of T, B, and NK cells. These observations lead to lowering the immune response in cell transplantation in diabetes and neurogenerative diseases, skin implants, and other illnesses [18]. However, defects in Sertoli cells can cause infertility. Alternately, Oct4, a pluripotent transcription factor, is involved in maintaining self-renewal in stem cells and somatic cells [19, 20]. In this context, SF1 is critical in regulating the transcription of Oct4. However, NR5A2 (LRH-1) can program somatic cells, leading to iPSCs, and thus can replace Oct4 and result in a better yield of iPSCs [21]. The substantial fold increases of NR5A1 is of enormous significance for the reproduction and survival of the human species. We did not detect SHP (NROB2) expressed in fibroblasts but it is down regulated in stem cells. [22]. normal cells, such as embryonic stem cells or early embryos, down-regulation of SHP-1 can also affect the expression of Nanog, a key pluripotency factor that maintains the self-renewal and differentiation potential of these cells. Nanog expression is regulated by STAT3 phosphorylation, which is negatively controlled by SHP-1 (23) . Therefore, down-regulation of SHP-1 can increase STAT3 phosphorylation and Nanog expression, which enhances the pluripotency and reprogramming of these cells. This can have implications for regenerative medicine and stem cell. Another possible explanation is that SHP gene transcription is constantly induced by the expression and activation of nuclear factor-erythroid two (NRF2), which encodes NFE2L2 [24]. In addition, NRF2 is vital in controlling the adverse effects of electrophilic and oxidative stress [23] and does so by activating the expression of a wide array of antioxidant response genes. On the one hand, applying NRF2 activators in clinical trials [24] could prevent cancer and treat some diseases related to oxidative stress but on the other hand, integral triggering of NRF2 in many cancers can lead to the survival and proliferation of tumor cells, as well as resistance to anticancer therapy [26]. [27] revealed that the NRF2 signaling pathway can be regulated and inhibited with NRs such as RARα, RXRα, PPARγ, ERα, ERRβ, and GR [28]. These Nuclear receptors are expressed by both stem cells and fibroblasts, and we see SHP is downregulated in stem cells and not expressed in fibroblasts. The expression of AHR is increased in skin fibroblasts (10-fold increase) compared to stem cells (60% downregulated). AHR [29,30], is involved in the function of the skin as the first barrier against many pathogens and environmental threats. Moreover, AHR controls [31] immune-mediated skin reactions and many physiological functions by acting as a sensor that mediates environment–cell interactions, predominantly during immune and inflammatory responses. Consequently, AHR plays a crucial role in skin integrity and immunity [32] in homeostasis and disease. This might explain the increased AHR expression. To further understand the direct interactions among NRs, we analyzed gene networks using Pathway Studio [33]. Nuclear receptor interaction maps showed the presence of a closed-loop network, as shown in Figure 1. The 25 most significantly enriched cell processes regulated by the gene set (p
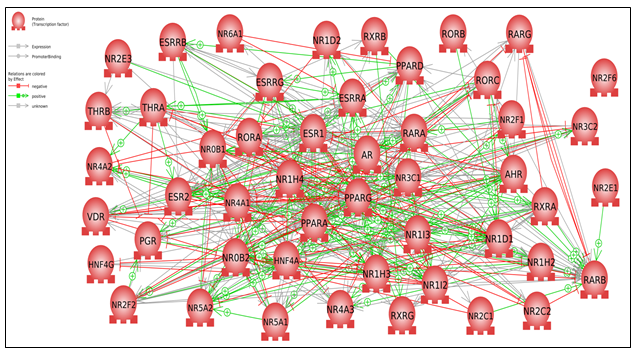
Figure 1: Feedback-loop Network Nuclear Receptor Interactions
Table 4: Cell processes regulated by the nuclear receptors
![]() |
Conclusion
Metadichol, is potentially a natural ligand for all 49 NRs. This result has a profound impact on the regulation of many biological processes, such as development, metabolism, reproduction, inflammation, and circadian rhythm. Previously we have shown that it binds to VDR, AHR, THRA, THRB, and RORC [12, 34, 35, 36]. . It is not therefore surprising that Metadichol ® effects multiple pathways in diseases. Cholesterol, long chain lipid alcohols like Metadichol
(a mixture of C26-C28-C30 alcohols (C28 is major constituent > 80%), fatty acids, fat-soluble vitamins, and other lipids in our meals are critical nutrients and precursors to nucleus receptor-binding ligands [37, 38 ]. To the best of our knowledge, this research is the first to show that NRs can be expressed from undifferentiated and differentiated cells. Our procedure is generic and can be applied to study variety of cells. NR’s are differentially expressed in the two groups of cells stems and fibroblasts. Studies of normal and diseases cells could reveal the role that nuclear receptors play and lead to a better understanding of diseases processes. More studies need to be carried out with different types of cells to better understand the nature of nuclear expression by treatment with Metadichol. Ongoing research based on this approach will be reported soon.
Declaration of Interest
The author is Founder and sole stake holder of privately held company Nanorx Inc NY 10514, USA. The author was involved with study, design data collection and analysis. The author declares no other competing interests.
Funding
R&D budget of Nanorx Inc
Availability of data and materials
All data are presented in manuscript and in the supplementary materials.
Supplementary materials Consist of the following Files
- Raw RT-PCR data of Regulation of Nuclear receptor genes in HMSC cells treated with Metadichol
- RAW RT-PCR Regulation of Nuclear Receptor genes in NHDF cells treated with Metadichol.
- Western Blot studies of Nuclear Receptor Protein expression using HMSC cells
- Western Blot studies of Nuclear Receptor Protein expression using NHDF-C adult cells
- Cell Processes regulated and enriched by Nuclear Receptors.
References:
- McEwan IJ and Kumar R. Nuclear Receptors: From Structure to the Clinic (Springer International Publishing) (2015).
- Frigo DE, Bondesson M, and Williams C. Nuclear receptors: from molecular mechanisms to therapeutics. Essays. Biochem 65 (2021): 847-856.
- Sladek FM. What are nuclear receptor ligands? Mol. Cell. Endocrinol 334 (2011): 3-13.
- Li J and Buchner J. Structure, function and regulation of the hsp90 machinery. Biomed. J 36 (2013), 106-117.
- Pike JW and Meyer MB. The vitamin D receptor: new paradigms for the regulation of gene expression by 1, 25-dihydroxyvitamin D3. Endocrinol. Metab. Clin. North. Am 39 (2010): 255-269.
- Burris TP, Solt LA, Wang Y, et al. Nuclear receptors and their selective pharmacologic modulators. Pharmacol. Rev 65 (2013): 710-778.
- Biddie SC and John S. Minireview: conversing with chromatin: the language of nuclear receptors. Mol. Endocrinol 28 (2014): 3-15.
- Xie CQ, Jeong Y, Fu M, et al. Expression profiling of nuclear receptors in human and mouse embryonic stem cells. Mol. Endocrinol 23 (2009): 724-773.
- Klemm SL, Shipony Z and Greenleaf WJ. Chromatin accessibility and the regulatory epigenome. Nat. Rev. Genet 20 (2019): 207-220.
- Hong SH, Nah HY, Lee YJ, et al. Expression of estrogen receptor-α and -β, glucocorticoid receptor, and progesterone receptor genes in human embryonic stem cells and embryoid bodies. Mol. Cell 18 (2004): 320-325.
- Raghavan PR. Metadichol® a nano lipid emulsion that expresses all 49 nuclear receptors in stem and somatic cells (2022-1)
- Raghavan PR. Metadichol liquid and gel nanoparticle formulations. US patent 9,006,292 B2 (2015).
- Miller WL and Auchus RJ. The molecular biology, biochemistry, and physiology of human steroidogenesis and its disorders. Endocr Rev 32 (2011): 81-151.
- Liang J, Wang N, He J, et al. Induction of Sertoli-like cells from human fibroblasts by NR5A1 and GATA4. ELife 8 (2019): 48767.
- Rotgers E, Jørgensen A and Yao HHC. At the crossroads of fate-somatic cell lineage specification in the fetal gonad. Endocr. Rev 39 (2018): 739-759.
- Kaur G, Thompson LA and Dufour JM. Sertoli cells–immunological sentinels of spermatogenesis. Semin. Cell Dev. Biol 30 (2014): 36-44.
- Kaur G, Thompson LA and Dufour JM. Therapeutic potential of immune privileged Sertoli cells. Anim. Reprod 12 (2015): 105-117.
- Mital P, Kaur G and Dufour JM. Immunoprotective Sertoli cells: making allogeneic and xenogeneic transplantation feasible. Reprod 139 (2010): 495-504.
- Luca G, Arato I, Sorci G, et al. Sertoli cells for cell transplantation: pre-clinical studies and future perspectives. Andrology 6 (2018): 385-395.
- Yang HM, Do HJ, Kim DK, et al. Transcriptional regulation of human Oct4 by steroidogenic factor 1. J. Cell. Biochem 101 (2007): 1198-1209.
- Heng JCD, Feng B, Han J, et al. The nuclear receptor Nr5a2 can replace Oct4 in the reprogramming of murine somatic cells to pluripotent cells. Cell Stem Cell 6 (2010): 167-174.
- Huang J, Tabbi-Anneni I, Gunda V, et al. Transcription factor Nrf2 regulates SHP and lipogenic gene expression in hepatic lipid metabolism. Am. J. Physiol. Gastrointest. Liver Physiol 299 (2010): 1211-1221.
- Han Y, et al, 2006. Loss of SHP1 enhances JAK3/STAT3 signaling and decreases proteosome degradation of JAK3 and NPM-ALK in ALK+ anaplastic large-cell lymphoma. Blood. (2006) Oct;108(8) 2796-2803.
- Xue D, Zhou X and Qiu J. Emerging role of NRF2 in ROS-mediated tumor chemoresistance. Biomed Pharmaoother 131 (2020): 110676.
- Yagashita Y, Gatbonto Schwager TN, McCallum ML, et al. Current landscape of NRF2 biomarkers in clinical trials. Antioxidants 9 (2020): 716.
- Dornas W, Sharanek A and Lagente V. Nuclear factor (erythroid derived 2)- like 2/antioxidant response element pathway in liver fibrosis. Reactive Oxygen species 7 (2019): 64-67.
- Akhileshwar Namani, Yulong Li, Xiu JunWang, et al. Modulation of NRF2 signaling pathway by nuclear receptors: Implications for cancer. Biochimica et Biophysica Acta 1843 (2014): 1875-1885.
- Raghavan PR. Metadichol, a natural ligand for expression of Yamanaka reprogramming factors in somatic and primary cancer cell lines (2022).
- Nakatsuji T and Gallo R L. The role of the skin microbiome in atopic dermatitis. Ann. Allergy Asthma Immunol 122 (2019): 263-269.
- Fernández-Gallego N, Sánchez-Madrid F and Cibrian D. Role of AHR ligands in skin homeostasis and cutaneous inflammation. Cells 10 (2021): 3176.
- Roztocil E, Hammond CL, Gonzalez MO, et al. The aryl hydrocarbon receptor pathway controls matrix metalloproteinase-1 and collagen levels in human orbital fibroblasts. Sci. Rep 10 (2020): 1-16.
- Rothhammer V and Quintana FJ. The aryl hydrocarbon receptor: an environmental sensor integrating immune responses in health and disease. Nat. Rev. Immunol 19 (2019): 184-197.
- Nikitin A, Egorov S, Daraselia N, et al. Pathway studio--the analysis and navigation of molecular networks. Bioinformatics 19 (2003): 2155-2157.
- Raghavan P.R. Metadichol ®. A novel inverse agonist of Aryl Hydrocarbon Receptor (AHR) and NRF2 inhibitor. J. Cancer. Sci. Ther 9 (2017): 661-668.
- Raghavan P.R. Metadichol, a novel ROR gamma inverse agonist and its applications in psoriasis. J. Clin. Exp. Dermatol. Res 8 (2017-1): 433.
- Raghavan P.R. Metadichol® A novel inverse agonist of thyroid receptor and its applications in thyroid diseases. Biol. Med 11 (2019): 2.
- Chawla A, Repa JJ, Evans RM, et al. Nuclear receptors and lipid physiology: opening the X-files. Science (New York, N.Y.) 294 (2011): 1866-1870.
- Thorne, J.L., Cioccoloni, G . Nuclear Receptors and Lipid Sensing (2022). In: Campbell, M.J., Bevan, C.L. (eds) Nuclear Receptors in Human Health and Disease. Advances in Experimental Medicine and Biology, vol 1390. Springer, Cham. https://doi.org/10.1007/978-3-031-11836-4_5