Toxicity of SARS-CoV-2 Spike Protein from the Virus and Produced from COVID-19 mRNA or Adenoviral DNA Vaccines
Article Information
Jean-François Lesgards*, 1, Dominique Cerdan2, Christian Perronne3, Jean-Marc Sabatier4, Xavier Azalbert5, Elizabeth A. Rodgers6, Peter A. McCullough7
1Université Aix-Marseille, Ingénierie des peptides thérapeutiques, Ambrilia-Cellpep, Faculté de Médecine, Boulevard Pierre Dramard, 13015 – Marseille, France
2Immunology, Université Victor Segalen, 3 Place de la Victoire, 33000 Bordeaux, France
3Infectious and tropical diseases, Paris, France
4Université Aix-Marseille, Institut de Neuro-Physiopathologie (INP), UMR 7051, Faculté de Pharmacie, 27 Bd Jean Moulin, 13385, Marseille Cedex, France
5Ecole d'Economie de Toulouse - TSE, 1988 Econometrics, France
6Department of Epidemiology, Graduate School of Public Health, University of Pittsburgh, 4200 Fifth Ave, 15260 Pittsburgh, USA
7Chief Medical Advisor Truth for Health Foundation, Post Office Box 64507, 85728 Tucson, AZ
*Corresponding author: Jean-François Lesgards, Université Aix-Marseille, Ingénierie des peptides thérapeutiques, Ambrilia-Cellpep, Faculté de Médecine, Boulevard Pierre Dramard, 13015 – Marseille, France.
Received: 10 July 2023 Accepted: 19 July 2023 Published: 28 July 2023
Citation: Jean-François Lesgards, Dominique Cerdan, Christian Perronne, Jean-Marc Sabatier, Xavier Azalbert, Elizabeth A. Rodgers, Peter A. McCullough. Toxicity of SARS-CoV-2 Spike Protein from the Virus and Produced from COVID-19 mRNA or Adenoviral DNA Vaccines. Archives of Microbiology and Immunology. 7 (2023): 121- 138.
View / Download Pdf Share at FacebookAbstract
COVID-19 has already killed millions of people around the globe through severe acute respiratory syndrome coronavirus 2 (SARS-CoV-2) infection followed by immuno-inflammation processes induced by viral proteins in particular the Spike protein. COVID-19 vaccines in particular mRNA and DNA vaccines have been proposed and used worldwide but they have been recognized to be associated with or being the cause of various side effects. Generally mild but also more severe side effects have been observed such as thrombosis, myocarditis, hepatitis, neurological side effects, and menstruation perturbations which are accessible and described in official registries (Vaccine Adverse Event Reporting System (VAERS- CDC, EudraVigilance). The purpose of this article is to summarize and discuss immuno-inflammatory and other biochemical mechanisms that may be common to both COVID-19 pathology and vaccination side effects using mRNA and DNA technologies which induce the human body’s production of a close replica of the spike protein of SARS-CoV-2. We propose that the main inflammatory pathway may be the activation of the complement system (lectin and alternate pathways). Others key toxic pathways are the activation of [des-Arg9] -bradykinin (ACE2/bradykinin B1R/DABK axis), dysregulation of the renin-angiotensin-aldosterone system as well as the attenuation of the Mas receptor activity (ACE2/MasR axis). Also the crucial importance of oxidative stress associated to inflammatory processes has been largely underestimated. Altogether this review indicates tracks for additional research efforts to identify more efficient and precise anti-inflammatory/antioxidant treatments against both COVID-19 and COVID-19 vaccine side effects.
Keywords
Spike protein - COVID-19 Vaccines side effects – Immuno-Inflammation – Lectin pathway – Bradykinin – renin angiotensin aldosterone
Spike protein articles, COVID-19 Vaccines side effects articles, Immuno-Inflammation articles, Lectin pathway articles, Bradykinin articles, renin angiotensin aldosterone articles
Article Details
MeSH Keywords:
Spike protein - SARS-CoV-2 - COVID-19 - COVID-19 vaccine - Inflammation – Blood - Coagulation - Microglia
1. Introduction
The spike protein allows SARS-CoV-2 to anchor itself onto human cells via its receptor Angiotensin-converting enzyme 2 (ACE2) which is a membrane-bound peptidase [1]. Subsequently, the spike protein is cleaved by the transmembrane protease serine 2 (TMPRSS2) to mediate virus fusion with the cell membrane and its uptake [2]. ACE2 is a key protein for our health. Indeed it is an enzyme which has crucial roles for the human organism: it helps to regulate blood pressure through the renin angiotensin aldosterone system (RAAS), and it is essential to fight inflammation in our body, which is particularly key in the pathology of COVID-19. When ACE2 is occupied by its link with the virus, it can no longer play its essential anti-inflammatory role. In particular that induces an imbalance with another enzyme with which ACE2 is normally in equilibrium, the Angiotensin-converting enzyme (ACE), which activates inflammation and which is left free by the virus. This ACE2/ACE imbalance participates to the hyper- inflammation in COVID-19 that develops through major biochemical pathways described in this review. By binding to ACE2 the spike protein of the virus triggers a significant part of the inflammation, the so called “cytokine storm”, pneumonia and potential acute respiratory distress syndrome (ARDS) with clotting, and finally death. It is this very closely the same spike protein that mRNA and DNA vaccines produce in large and uncontrolled quantities in the body and that may have similar affinity for ACE2 receptor [3, 4]. Therefore, the spike protein from the vaccines in particular in its free form, is potentially able to trigger the same inflammatory processes as the virus spike protein. The inflammatory toxicity of spike protein has been reported and well known in the scientific literature for at least ten years. The second factor which increases the toxicity of spike/ACE2 interaction is that ACE2 receptors are present almost everywhere in the body: pharynx, trachea, lungs, blood, heart, vessels, intestines, brain, male genitalia and kidneys, and also in body fluids (mucus, saliva, urine, cerebrospinal fluid, semen and breast milk) [5].
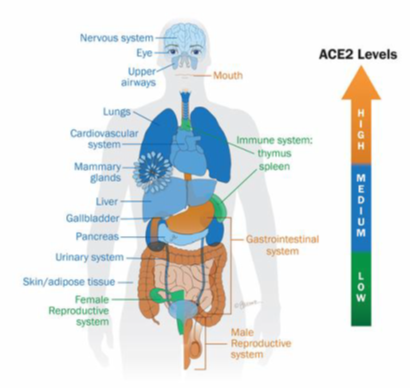
Figure 1: Overview of the expression level of ACE2 receptors
The color gradient from orange to green indicates high or low level of ACE2 expression in tissues and body fluids. The highest levels are detected in the oral cavity, gastrointestinal tract and male reproductive system [5].
As a result, spike protein is able to set off inflammation in many organs and systems. Indeed, the majority of patients with COVID-19 have various other symptoms in addition to respiratory disorders, including neurological, cardiovascular, intestinal and renal dysfunctions [6, 7, 8, 9, 10]. Since spike protein is present in SARS-CoV-2 (and its variants) and produced by the vaccines, this toxicity therefore applies both to COVID-19 (severe forms and also long COVID) but also potentially to all vaccines which are based on the uncontrolled production of this spike protein by various cells versus vaccines which are made from deactivated whole virus or based on deactivated spike protein. It is known that after injection with mRNA vaccines, spike protein is found both on the surface of the cells (where the manufacturers have told it would be found) but also in significant quantities under free form circulating in blood thus reaching different organs including the heart, brain, liver, kidneys, and the brain [11]. Moreover, it has been demonstrated that the whole spike protein alone as well as the S1, which contains the ACE-2 receptor-binding domain (RBD), can interact with ACE2 receptors expressed by various cells, including platelets and endothelial cells, inducing inflammatory processes [11,12]. Therefore, it is imperative that the vaccine companies give complete information not only on the toxicity of mRNA encapsulated in lipid nanoparticles (LNPs) but also of vaccine spike protein.
2. Description of spike protein-induced immuno-inflammatory processes and associated biochemical pathways
The main hallmark of severe cases of COVID-19 is pneumonia, with potential acute respiratory distress syndrome (ARDS) that requires patients to be treated in intensive care unit. After the replication phase of the virus a strong inflammation occurs. Fifteen percent of patients develop dysfunctional and harmful procoagulant and inflammatory disease, which results in life-threatening respiratory distress. The famous "cytokine storm" is an uncontrolled release of cytokines that has been observed in some infectious and non-infectious diseases. But the term is often used, including by many physicians and researchers, without sufficiently specifying the biochemical pathways involved which impacts negatively the search of more precise potential therapies. The spike protein allows great dissemination of the virus through the epithelia and then through the bloodstream to various organs. This is also the case for the vaccines. In these organs and vessels, spike proteins can spread inflammation by the 4 main following pathways (figure 2):
- Dysregulation of the renin-angiotensin-aldosterone system (ACE/angiotensin II / AT1R axis)
- Attenuation of the Mas receptor activity (ACE2/MasR axis)
- Activation of [des-Arg9] -bradykinin (ACE2/bradykinin B1R/DABK axis)
- Activation of the complement system (lectin and alternate pathways) including components C5a and C5b-9
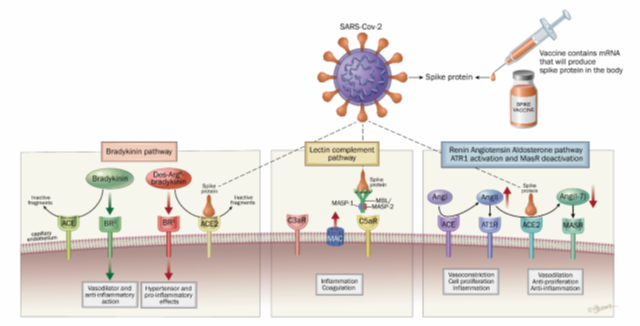
Figure 2: Main inflammatory pathways explaining the toxicity of SARS-CoV-2 spike protein with respiratory infection and produced from COVID-19 mRNA and adenoviral DNA vaccines.
2.1 Dysregulation of the renin-angiotensin-aldosterone system (ACE/angiotensin II / AT1R axis)
The renin-angiotensin-aldosterone system (RAAS) is, in mammals, one of the most important regulatory systems for autonomic, cardiovascular, and pulmonary functions. It is a hormonal system organized around the kidney, which makes it possible to preserve hydrosodium homeostasis. The RAAS regulates blood pressure and also plays an important role in inflammation [14]. As mentioned previously, ACE2 protein plays a beneficial role: vasodilator, antioxidant, and anti-inflammatory while its counterpart, the ACE protein is on the contrary vasoconstrictor, pro-oxidant, and pro-inflammatory. ACE2 plays this role by controlling the amount of an essential peptide, angiotensin II produced by ACE from another small protein or peptide, angiotensin I. Angiotensin I is produced by the kidney as a result of the enzyme renin from the angiotensinogenic peptide which is formed in the liver and released into the plasma. As ACE2 is depleted because of the presence and multiplication of the virus, the balance tilts in favor of the inflammation produced by angiotensin II which is no longer degraded through the numerous mediators that result from it especially in the lungs. This inflammation occurs through the interaction between angiotensin II and the AT1 receptor (Angiotensin II type 1 receptor, AT1R) in the kidney and the vascular system [15, 16]. A significant production of cytokines results from this, such as the growth factor TGF-β. Angiotensin II can then activate the pivotal pathway of nuclear factor kappa B (NF-κB), a central component of the inflammatory response, which is also associated and induced by other important cytokines in COVID-19: IL-1B , IL-6, TNFα, IL-10, MCP1 (monocyte chemoattractant protein 1), AT1 and platelet-derived growth factor beta (PDGF-B) [17, 18, 19].
2.2 Attenuation of the Mas receptor activity (ACE2/MasR axis)
The ACE2/MasR receptors and Ang- (1–7) are the constituents of the other arm of the RAAS system which counteracts and attenuates the effects of the first axis ACE-Ang II-AT1R described above and illustrated in Figure 3 [20].
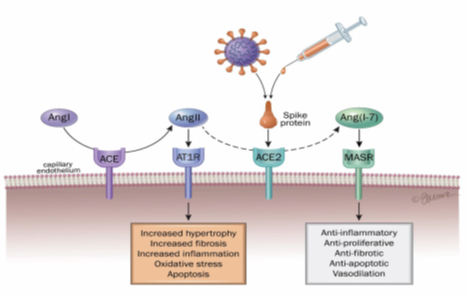
Figure 3: Schematic overview of RAAS perturbation by spike protein (virus or vaccine spike)
Angiotensinogen is secreted by the liver and is converted to angiotensin I (AngI) via renin, a protease produced in the kidneys. AngI is then converted to AngII by the catalytic action of angiotensin converting enzyme (ACE) and binds to angiotensin II type 1 (AT1) and type 2 (AT2) receptors. AngII binds to the angiotensin type 1 receptor (AT1R) to promote actions such as vasoconstriction, cell proliferation, fibrosis, and inflammation. ACE2 converts Ang-I and Ang-II to angiotensin (1–7). Ang (1–7) binds to the MAS receptor (MASR) to promote vasodilation, vascular protection, anti-fibrosis, anti-proliferation, anti-inflammation and anti-angiogenesis actions [20].
This is an axis of protection against inflammation which is weakened due to the monopolization of the ACE2 receptor by the virus. The beneficial effect of ACE2 occurs through the cleavage of angiotensin II into the Ang- (1–7) peptide. It is the latter molecule which has a vasodilator effect, antiproliferative (anti-cancer), anti-thrombotic and anti-inflammatory properties and is therefore found to be in deficit. Ang- (1–7) reduces the expression of inflammatory factors such as NF-κB, IL-6, TNFα and IL-8 [21, 22, 23].
2.3 Activation of [des-Arg9] -bradykinin (ACE2/bradykinin B1R/DABK axis)
Infection with SARS-CoV-2 by depleting ACE2 also increases the levels of des-Arg9-bradykinin (DABK) which is a known pulmonary inflammatory peptide factor. DABK is a bioactive metabolite of bradykinin which is associated with lung damage and inflammation, primarily through bradykinin receptor 1 (BR1) receptors in the endothelial cells of the lungs. The kinin-kallikrein system includes kininogen, the enzyme kallikrein, bradykinin (BK-1–9 or BK) and DABK (figure 4). ACE2 participates in the degradation of DABK, a process ultimately by the downregulation of ACE2 induced by the virus and thus leading to a loss of control of this inflammatory pathway [24]. Crosstalk between angiotensin, the kinin-kallikrein system, as well as the inflammatory and coagulation systems play a critical role in COVID-19. Cardiac complications and coagulopathies involve crosses between these systems and should be a more tested route of study to prevent or control ARDS in patients with severe COVID-19 [25, 26, 27].

Figure 4: Action of ACE and ACE2 enzymes in the KKK system (kininogen-kinin-kallikrein)
- ACE degrades bradykinin, a vasodilator peptide acting primarily through BR2 receptors. Bradykinin can also be converted by kininase I to des-Arg9-bradykinin (DABK), which promotes vasoconstriction and pro-inflammatory effects upon interaction with BR1 receptors.
- SARS-CoV2 mediated imbalance in the KKK system with predominant pro-inflammatory effect of des-Arg9-bradykinin [27].
A review of the literature suggests that the control of the bradykinin pathway may be a feasible option in the management of COVID-19 in patients with vascular pathologies and that a supportive treatment of respiratory complications and cardiology is necessary in COVID-19 patients, in particular by C1-inhibitors (C1-INH) [26, 28, 29]. This inflammatory pathway appears to be much more important in COVID-19 than the first two described.
2.4 Activation of the complement system (lectin and alternate pathways) including components C5a and C5b-9
The complement system contributes to the innate immune response which includes the cells and mechanisms immediately allowing the body's defense against infectious agents in the absence of antibodies. It does not require cell division, conversely of the adaptive immune system which confers a later but more durable protection and which requires cell division (lymphocytes B and T). Its excessive activation during COVID-19 is involved in cytokine storm, endothelial inflammation, and the thrombosis that accompany the disease in the most severe forms and death.
There are three biochemical pathways that activate the complement system: the classical complement pathway, the alternate complement pathway, and the lectins pathway binding mannose and N-acetylglucosamine residues of the sugars present in bacterial and viral membranes. The involvement of the complement system in the pathogenesis of infection by syncytial virus, MERS-CoV and SARS-CoV-1, is already known from several studies [30, 31, 32]. Hyperactivation of complement components includes C5a in serum and C5b-9 in lungs was observed in hDPP4 transgenic mice infected with MERS-CoV. Lung and spleen induced damage and inflammatory responses were attenuated by blocking the C5a - C5aR axis in these transgenic mice [33].
The lectin pathway is a priority pathway in COVID-19 [32]. It is activated by the binding of a mannan-binding lectin (MBL)-mannan-binding lectin serine protease 2 (MASP-2) complex to the surface of a pathogen. This complex, linked to the pathogen, then cleaves C4 and C2. These cleavages form a C3 convertase (C4b2a). Studies and literature reviews highlight the role of MASP binding to SARS-COV-2, activation of the lectin pathway, and blood coagulation, as well as their associations with COVID-19 comorbidities. Therefore, it is likely a key pathway linking inflammation and the end-stage coagulation phenomena of severe forms of COVID-19. Although N protein activates the lectin complement pathway, the spike protein has also been shown to activate this pathway [34, 35, 36, 37]. Of important note, different molecules of the biochemical lectins pathway were found in the lung tissues recovered during autopsies of subjects who were reported to die of COVID-19: MBL, MASP-2, C4alpha, C3 or C5b- antibody 9 [30].
Lectin-type receptors (CLRs), in particular dendritic cell-specific intercellular adhesion molecule-3-Grabbing non-integrin (DC-SIGN) (and also liver/lymph node-specific intercellular adhesion molecule-3-grabbing integrin [L-SIGN], mannose receptor [MR], and macrophage galactose lectin [MGL]), on the surface of cells of the innate immune system (such as macrophages and dendritic cells) can direct immune responses of the host against the virus (S proteins in particular) by identifying specific sugars (glycans: oligomannose N-glycans) associated with virus proteins [38].
Viral inactivation by the complement cascade involves absorption and clearance of the virus by phagocytosis, which prevents attachment to their receptors, lysis of the virus by the formation of pores, and destruction of its membrane by formation of a membrane attack complex (C5b-9) (MAC) [39]. The increase in Ang II production and the activation of AT1R are accompanied by a pro-inflammatory response via the activation of the complement cascade comprising C5a, and C5b-9. Complementary factor 5a (C5a) is the most powerful inflammatory peptide in the complement cascade which induces the release of numerous pro-inflammatory cytokines including IL-1, IL-6, TNF-α, NF-κB, activator Protein-1 (AP-1) [40]. In addition, increased production of C3a leads to the production of pro-inflammatory cytokines such as IL-1, IL-6 and TNF-α. If the virus has not been cleared by the immune system at the epithelial barrier, it will then progress to vascular endothelium which express ACE2 receptors.
Viral penetration into these cells will cause endothelial inflammation called endothelitis or endothelialitis [41]. Endothelitis is associated with the thrombotic phenomena observed in COVID-19, such as microthrombosis and macrovascular thrombosis, as well as pulmonary embolism [42, 43]. The complement has been associated with endothelitis lesions seen in severe forms of COVID-19. Histological deposits of MASP-2, C4 and MAC, as well as macrophages overexpressing the C5a receptor, C5aR1, have in fact been found in endothelitis lesions and microthrombi [44]. The complement and coagulation (contact phase, or intrinsic pathway of coagulation) are closely linked [45]. Indeed, MASP-1 and MASP-2 have a catalytic role for prothrombin and fibrinogen, leading to thrombotic complications (figure 5) [46, 47]. Finally, MASP 1 and/or 2 have increased expression in patients with risk factors for COVID-19: diabetes, high blood pressure and cardiovascular disease, chronic kidney disease, chronic obstructive pulmonary disease and cerebrovascular disease [33].

Figure 5: Lectin-complement pathway induced SARS-CoV2 and mRNA vaccines spike protein
The lectin pathway is activated by the binding of an MBL (mannan-binding lectin)-MASP-2 (mannan-binding lectin serine protease 2) complex to the surface of a pathogen. This complex, linked to the pathogen, then cleaves the C4 and C2. These cleavages form a C3 convertase (C4b2a). Activation of the classical pathway is initiated by binding of the C1 complex to immunoglobulins or endogenous ligands. This complex will be able to cleave C4 and C2 to form the classic C3 convertase (C4b2a) which cleaves the C3 into C3a, an anaphylatoxin, and into C3b, causing the formation of C5 convertase (C4b2a3b or C3bBb3b). The C5 is then cleaved into C5a and C5b, which will initiate the final stages of the complement cascade. This leads to formation of the membrane attack complex (C5b-9, MAC) which allows pathogens to be lysed.
3. A comparison of spike protein-induced toxicity in the setting of SARS-CoV-2 infection versus injections of COVID-19 mRNA and adenoviral DNA vaccines. Proposal of biochemical mechanisms
As mentioned before, during COVID-19, the immuno-inflammatory pathways occur not only in lung, but also in all organs that contain ACE2 receptors [5]. This is also potentially the case for the spike protein generated in high amounts in the body and of which a part has been found to circulate free in the circulation [11]. Therefore, it is justified to investigate more deeply and to propose mechanisms for both COVID-19 disease and vaccine side effects in all the organs where they have been reported. Severe side effects are difficult to assess and there is currently no consensus on the exact counts. Of note, the US VAERS database provide a signal that appears significant and sometimes associated mortality which may be largely underestimated. Indeed, it is estimated that the reports of side effects represent only about 10% of reality [48].
3.1 Damage on vessels and cardiovascular system
Lei et al. (2021) showed that using a pseudo-virus expressing S protein (pseudo-spike devoid of viral RNA), specifically the S1 part which contains the RBD of SARS-CoV2 caused inflammation and damage in the arteries and lungs of mice exposed intratracheally [49]. The same phenomenon has been observed on human epithelial cells resulting in attack of the mitochondria. The work of these researchers clearly shows that the spike protein alone, not associated with the rest of the viral genome, is sufficient to cause the cardiovascular damage associated with COVID-19. This suggests that both SARS-CoV-2 and free spike released after COVID-19 vaccination could result in the same cardiovascular damages. Moreover, in an in vitro study, researchers showed that spike alone (S1 part) induced a loss of integrity of the blood-brain barrier (which separates the vessels supplying the brain from the central nervous system) on a model reconstructing this barrier, suggesting the possibility of inflammation in brain vessels [50]. For vaccines, in addition to the free spike found in the circulation, the spike protein can be expressed on endothelial cells and can activate blood platelets and coagulation, which also leads to thrombosis (by releasing platelet factor 4 or PF4) [51, 52].
Thrombosis after vaccination have been described, as well as myocarditis and pericarditis, stroke, in association high levels of D-dimer (coagulation marker) and CRP (C-reactive protein: inflammation marker) [52, 53, 54]. Of note, in a post-vaccination study with the AstraZeneca vaccine, thromboses were observed on young subjects (between 22 and 49 years of age, mean age 36 years) whereas severe COVID-19 occur essentially in much older people and elderly [52]. They developed venous thromboses, including nine patients with cerebral thrombosis, three with pulmonary embolism and six of the eleven patients died. When circulating virus and spike proteins for vaccine react with the ubiquitous ACE2 receptors in the body this upset the ACE2/ACE balance at these sites [3, 55]. It is known that in almost all pathological conditions, in particular those involving the cardiovascular system but also neurodegenerative damages, there is a decrease in the ACE2/ACE ratio within the organs [56, 57, 58, 59, 60].
Some cases of sudden cardiac deaths have also been described. The same four mechanisms highlighted in this review (including RAAS, the complement pathway and bradykinin) could be partly implicated, but we also propose here the hypothesis that a rupture of the cardiac syncytium could occur especially after various injections. As it has been proposed and shown for a few COVID-19 cases, spike protein could drive cell-cell membrane fusion between cardiomyocytes that would lead to aberrant electrophysiological activity, electromechanical dysfunction, and fatal arrhythmia [61, 62]. In a young woman who died suddenly and was diagnosed post-mortem with COVID-19, some authors have observed highly focal myocardial SARS-CoV-2 infection spreading from one cardiomyocyte to another, through intercellular junctions identified by highly concentrated sarcolemmal t-tubule viral spike glycoprotein [61].
The formation of syncytial multinucleated giant cells facilitated by S2 spike domain could lead ultimately to irreversible electrophysiological dysfunction and sudden cardiac arrest [63, 64, 65]. In the case of vaccines it could be facilitated by the presence of LNPs [66].
Another hypothesis could be the formation of amyloid structure. It has been proposed that severe inflammatory disease including ARDS in combination with SARS-CoV-2 protein aggregation might induce systemic AA amyloidosis [67]. Cases which showed amyloid deposits in vessels shortly after vaccination have been described [68]. Furthermore several sequences of spike protein of around 20 amino-acids (192−211, 601−620, 1166−1185) fulfill amyloid fibril criteria [69]. Neutrophil elastase which is overexpressed on sites of inflammation of viral infection, cleaved spike protein, thus permitting the exposure of amyloidogenic segments.
3.2 Neurological toxicity and damages
SARS-CoV-2 is not only a respiratory virus but is in fact multi-target. In particular, it has a high tropism for the central nervous system (CNS) as shown by several previous studies [70, 71, 72]. A study covering 214 COVID-19 patients, reported that 78 (36.4%) had neurological manifestations which included malaise, headache, and loss of smell (anosmia) and taste (dysgueusia) as well as more serious complications, such as ischemic stroke associated with significantly higher mortality [70, 73]. SARS-CoV-2 is also detected in the brain of a majority of examined patients [71]. ACE2 receptors are expressed in the human CNS, particularly in the spinal cord, spinal ganglion, brainstem nigra, choroid plexuses, hypothalamus, hippocampus, middle temporal gyrus, and posterior cingulate cortex [74, 75].
The virus has been shown to pass through olfactory or vagus nerve, as well as the blood–brain barrier (BBB) [70, 74, 76]. Another route is the blood-cerebrospinal fluid (CSF) barrier, which is a more permeable barrier compared to the BBB, formed by a single layer of endothelial cells of the choroid plexus [77, 78]. Inflammation induced by SARS-CoV-2 infection in the CNS seem to be linked to microglia activation and astrocytes inflammation [79]. Microglial activation has been observed in several studies including post-mortem studies [71, 80, 81]. After vaccination spike protein could also initiate inflammation in CNS through microglia and astrocytes, as well as the mechanisms described before (lectin-complement, bradykinin, and RAAS). It has been shown that mRNA and free spike protein can reach the brain through circulation and by crossing the BBB [50, 82].
Bahl et al. showed that mRNA of vaccines can reach the brain, as also specified by the European Medicines Agency (EMA) up to 2% of the mRNA plasma concentration [83, 84]. Other authors have shown that S1-induced neuro-inflammation in microglia mediated by the activation of NF-κB and p38 MAPK and increased release of TNF-α, IL-6 and IL-1β and NO [85]. Spike alone (S1 part) induced a loss of integrity of BBB on a model reconstructing this barrier, suggesting the possibility of inflammation in brain vessels and in the brain itself [50, 82]. Furthermore, activated microglia as well as activation of complement represent a common pathological feature of several neurodegenerative diseases, including Alzheimer's disease [86, 87, 88, 89].
Therefore, neurodegenerative disease in the global population needs to be watched closely regarding long COVID patients as well as the long-term side effects of COVID-19 vaccination [90]. The increase of BBB permeability though tight junction alterations observed in inflammatory conditions like diabetes should be taken into account during vaccination [91]. Additionally, the spike protein of SARS-CoV-2 and the vaccine induces a decrease in serotonin by mobilizing ACE2, which aggravates or can cause depressive or even suicidal states [91, 93]. Of note, anosmia has been described following vaccination in COVID-19 negative subjects. This shows that symptoms identical to COVID-19 can appear and have been triggered only by spike protein [94]. It is likely that the chronic condition characterized by fatigue and neuropsychiatric symptoms, termed long-COVID and severe CNS damage could be induced by microglia activity and inflammation in astrocytes induced by spike protein from SARS-CoV-2 as well as from spike produced by vaccine [95].
3.3 Liver
Liver is also a target of COVID-19. Regarding vaccines, after 48 hours (with maximum concentrations observed between 8 to 48 hours), mRNA is found mainly in the liver (up to 21.5%), the adrenal glands, the spleen (≤ 1.1%) and the ovaries (≤ 0.1%). Thus, the liver is the organ where mRNA concentrates the most after COVID-19 vaccine injections. Furthermore, circulating spike protein can also accumulate in the portal vein after vaccination, as it is a key passage in the body regarding the central role of liver. The spike-induced mechanisms of inflammation described in this review can contribute to thrombosis in the portal vein. It has been proposed that among cirrhotic patients infected with COVID-19, portal vein thrombosis may be a potential complication even in the absence of hepatocellular carcinoma [96]. Portal vein thrombosis occurring after mRNA and DNA injections has been described in several studies [97, 98, 99]. In particular mechanical aspiration revealed massive acute thrombi from the extrahepatic portal vein and the superior and inferior mesenteric veins. Control venograms showed recanalisation of the portal venous system with minimal residual thrombi in the superior mesenteric vein [98]. The patient had received the first dose of ChAdOx1 nCov-19 vaccination (AstraZeneca) 11 days previously. These patients need to be followed carefully, as it can induce portal hypertension as well as oesophageal varices that can be deadly. Other reports describe hepatitis following vaccination, potentially involving autoimmune reactions, some of which are fatal [100, 101, 102].
3.4 Menstruations problems
Menstruations problems have been described in COVID-19. But they seem to be more frequent after vaccination. Lee KMN et al. reported that 42% of women with regular menstrual cycles bled more heavily than usual post vaccination [103]. Excessive and/or prolonged menstruation with or without irregular bleeding (metrorrhagia and menorrhagia), loss of periods (amenorrhea) and worsening of endometriosis condition have been observed. An analysis on VAERS data from 1990 until November 12, 2021 found 14,331 reports on menstrual disorders (1.36% of total), of which 13,118 (90.90%) were exposed to COVID19 vaccine and 13,13 (9.10%) were exposed to another vaccine (ROR=7.83 for COVID-19 vaccines) [104]. Menstrual disorders were found increased particularly in the group of females aged 30–49 years, spiking to 97.4 % associated with COVID-19 vaccines among all other vaccines (vs 75 % for other ages), with a nearly tenfold increase in comparison with the pre-marketing period of the vaccine [104].
Various studies report changes after first dose between 25 % [105] and 50-60% of reproductive-age women [106] and up to 70 % after the 2nd dose [106]. Meanwhile these high values may be linked with some bias, for example when data were based on self-questionnaires from social media (LinkedIn, Facebook, and Twitter) among the general population, so women with menstrual issues could have been more motivated to answer than women who did not experience events [106]. The main sides effects were menorrhagia, metrorrhagia, and polymenorrhea (in 78,138 vaccinated females, 14 studies) [107] but also menstrual pain or cramps and abnormally heavy or prolonged bleeding [108, 109]. Also women with endometriosis seem more likely to experience changes in bleeding patterns (women with endometriosis: 39.5%, control group: 31.0%, p = 0.02) and a significant worsening in endometriosis-associated symptoms with an almost 4.3-fold worsening in dysmenorrhea [95% CI 1.9-9.9, p < 0.01] and 5.5-fold odds for any worsening in symptoms in endometriosis patients, as compared to the control group [95% CI 2.7-11.1, p < 0.01] [110]. In the majority of works, menstrual irregularities after both the first and second doses of the vaccine were found to self-resolve in approximately half the cases within two months. Meanwhile Zhang et al, in the VAERS study, also precise that only 18.01% of patients reported that their adverse reactions had vanished and 62.88% of patients reported that they still had adverse reactions related to vaccination [104].
Some authors suggest to consider these elements during the counselling of women who receive the COVID-19 vaccine which is mostly note done [106]. Regarding the mechanism of toxicity, menstrual cycle timing is regulated by the hypothalamic-pituitary-ovarian axis, which can be affected by life, environment, and health stressors. mRNA vaccines create a robust immune response or stressor, which could temporarily affect the hypothalamic-pituitary-ovarian axis [111]. The authors add that their results could not be explained by generalized pandemic stress because the unvaccinated control group showed no changes over a similar time period [111]. The spike protein receptor ACE2 is expressed in the ovaries, uterus, vagina, and placenta. Cytokines and glucocorticoids act on the hypothalamo-pituitary gonadal axis, arachidonic acid pathways, and the uterus, which leads to menstrual disturbances and pregnancy-related adverse events such as preterm labor and miscarriages [112]. Also, inflammation induced by spike protein from the virus or after vaccine injection, could occur directly in the ovaries and uterus as evidence suggests that ACE2 is widely expressed in the ovary, uterus, vagina and placenta [113]. After 48 hours following injection (with maximum concentrations observed 8 to 48 hours), mRNA is found mainly in the liver (up to 21.5%), the adrenal glands, the spleen (≤ 1.1%) but also in the ovaries (≤ 0.1%).
Thus, we propose that the inflammatory pathways detailed in this review could also occur through circulating spike proteins at microvascular level in both the ovaries and uterus, leading to menstrual perturbations. We also hypothesize that it could provoke the induction of the luteal phase therefore explaining shorter menstruations until arrest of cycles in some cases. More studies should observe, even retrospectively, if possible, the evolution of hormones levels and inflammation in women after both severe COVID-19 and vaccination. Such inflammation in severe COVID-19 has been shown to induce preeclampsia conditions [114] and it could also occur with vaccine-induced inflammation with sur-activation of complement pathway for example [115]. Finally, even if some concerns have been raised regarding spontaneous abortions among vaccinated women in early pregnancy, no significant enhancement have been described so far in publications using VAERS or other data bases [116].
4. The issue of antibody-dependent enhancement (ADE) in with SARS-CoV-2 and after COVID-19 vaccination
ADE is a phenomenon in which suboptimal neutralizing or non-neutralizing cross-reactive antibodies bind to virus and facilitate Fcγ receptor mediated enhanced entry into host cells, followed by its replication, and thus increasing the cellular viral load. The production of facilitating antibodies has been observed in many viral diseases and after vaccination for dengue fever, Zika, Ebola, HIV, SARS-CoV, MERS-CoV, and feline infectious peritonitis [117, 118, 119, 120, 121, 122, 123]. In the current context, if the antibodies that are acquired by people who are vaccinated with the current vaccines, based on the mRNA of the initial SARS-CoV-2 (February 2020) become ineffective in destroying the delta variant or future variants, then these variants could produce facilitator antibodies. As a result, infected people may often have a more serious form of illness.
The modeling work published by Yahi et al. shows that antibodies facilitating the spread of the virus (ADE) have more affinity with the spike protein than neutralizing antibodies with regard to the delta variant (on the contrary to what is observed with the original strain of SARS-CoV-2 of 2020, Wuhan/D614G) [124]. This work highlights the need of evaluating the neutralization/ADE balance of the variants following the initial virus in the sera of vaccinated individuals, especially in the frame of a massive vaccination campaign in order to adapt the vaccines to the most relevant and current variant [124]. The same team has also shown that a facilitating epitope located on the lower part of the N-terminal domain of the spike protein appeared to be highly conserved among most SARS-CoV-2 variants, which may represent a risk of antibody-dependent enhancement (ADE) [125]. Furthermore, ADE phenomenon occurs much more frequently with vaccines than with antibodies produced during an infection [126, 127]. Taken together, this could explain the observed ineffectiveness of COVID-19 vaccines against contamination and the very large number of vaccinated people who developed COVID-19 and died from it.
A few clinical studies have shown indications of ADE. According to Sridhar et al., ADE could be considered responsible for the aggravation of COVID-19 in the case of a woman recently vaccinated (7 days) with the mRNA BNT162b2 vaccine and who died from ARDS [128]. There was no trace of COVID-19 infection, however anti-spike antibodies were found on day 13 after injection. In another study, 2 patients with COVID-19 in the acute phase were vaccinated against COVID-19 with BNT162b2 vaccine (both on the 26th day of their COVID-19 illness) [129]. Both patients had COVID-19 pneumonia before receiving SARS-CoV-2 vaccination, and soon afterwards had new ground-glass opacities and respiratory failure. The authors suggest that the immune reaction to COVID-19 was reactivated by vaccination though they do not refer to ADE. Also, autopsies of 170 people who died from COVID-19 (or who were carriers of the virus at the time of death) showed lung viral loads much higher in the vaccinated group (n=29) than in the unvaccinated (n=141) (45% vs. 16%, respectively P = 0.008) and this was more significant in the partially vaccinated (n=16). The authors do not exclude the role of ADE in this phenomenon [130]. Finally, it also brings the discussion on the question of the safety of a large-scale vaccination during an epidemic or pandemic due to an RNA virus having a high propensity to mutate.
5. Specific inflammatory toxicity associated with mRNA vaccines
It is known that mRNA extracellular biomolecule can provoke inflammation, through toll-like receptors (TLR) and retinoic acid-inducible gene I (RIG-I) among other mechanisms, and predispose to endothelial damage, edema, hyper-coagulation and thromboembolic events, increases the permeability of the endothelial cells in brain [131] [132]. Furthermore before translation occurs, the mRNA may also bind to pattern recognition receptors (PRRs) within endosomes or cytosol and may also activate pro-inflammatory reactions such as inflammasome platforms, type I interferon (IFN) response and translocation of NF-κB [133].
Even if N1-methyl-pseudouridine presence in mRNA, should reduce its immunogenicity, this remain to be proven with more evidence [134] and very few mRNA vaccines have been tested on human subjects so far and only very recently [134]. In addition to mRNA and spike toxicity, of note is that the lipid nanoparticles (LNPs) are also known to cause clotting problems. This serious issue is present in a patent from Moderna on the injection of mRNA vaccine encapsulated in LNPs like in COVID-19 vaccines: “In some embodiments, the adverse effect includes coagulopathy, disseminated intravascular coagulation (DIC), vascular thrombosis, complement activation-related pseudo-allergy (CARPA), acute phase response (APR), or a combination thereof” [135].
This patent even provides for the addition of anticoagulants, antiallergics: “In some embodiments, the agent (molecule that can be added) inhibits platelet activation. In some embodiments, the agent is a platelet aggregation inhibitor. In some embodiments, the platelet aggregation inhibitor is aspirin or clopidrogrel (Plavix®). In certain embodiments, the platelet aggregation inhibitor is selected from aspirin/pravastatin, cilostazol, prasugrel, aspirin/dipyridamole, ticagrelor, cangrelor, elinogrel, dipyridamole and ticlopidine. In some embodiments, the agent inhibits CD36.” [135]. Clearly, inflammatory effects of both mRNA and NLPs can be common and be additive with those of spike-induced inflammatory processes, including the complement pathway and may contribute to severe side effects as well as COVID-19 conditions if vaccination occurs during COVID-19 infection or long COVID conditions [29, 136].
6. Discussion
The spike protein of SARS-CoV-1 is 76-78% identical to that of SARS-CoV-2 [137]. Toxicity of spike protein has been documented for more than ten years with SARS-CoV-1 [138]. In vivo studies have demonstrated that spike protein of SARS-CoV-1 worsens acute lung failure through inflammatory pathways similar to SARS-CoV-2 [139, 140]. Also, studies dating back to SARS-CoV-1 and MERS-CoV outbreaks, have shown that vaccines based on the whole spike protein could induce a strong immune inflammatory response in many organs and particularly in the lungs and liver [141, 142]. In these in vivo studies in ferrets, not only did vaccination fail to prevent infection, but vaccinated animals showed significantly stronger inflammatory responses than control animals and focal necrosis in liver tissue. These studies and others have shown that vaccinate against coronaviruses is very challenging [143].
A study by Pfizer shows that radioactive mRNA was detected in most tissues from the first moments after injection (15 minutes) and the results confirm that the injection site and the liver are the main sites of distribution [84]. Low levels of radioactivity were detected in most tissues, with the highest levels in plasma being observed one to four hours post dose. As previously referenced, at 48 hours mRNA is found mainly in the liver (up to 21.5%), the adrenal glands, the spleen (≤ 1.1%) and the ovaries (≤ 0.1%). It is imperative that additional research is done to evaluate precisely how long this toxic protein is produced and remains present in the cells of our organs but also in the bloodstream. It seems to be found after several weeks, with the possibility of producing chronic inflammation in many organs [3, 4, 5, 6, 83, 84]. The vaccines, which induce the production of spike protein, have a very strong inflammatory and oxidative potential over an undetermined period of time, given that the studies from the manufacturers have not yet provided this information. A study showed that the protein was found for at least 15 days after vaccination with Moderna, with a peak between one and five days at approximately 68 ng/L [3]. With the second injection (from 21 days) new spike proteins are produced, even as the antibodies and the body is working to eliminate this protein. Even if Ogata and his team observed a much shorter presence than for the first dose (a few days), there is potentially an inflammation which can be chronic and spread over several weeks, thus being able to destabilize the inflammatory balance in the blood vessels in the long term, the liver, brain, kidneys, etc. [3]. Röltgen K et al. have shown that mRNA vaccination stimulated robust germinal centers containing vaccine mRNA and spike antigen up to 8 weeks post vaccination in some cases [4]. Furthermore, the quantity of free spike protein in blood after COVID-19 vaccination has been found to be equivalent of that reached during an infection (up to 150 pg/ml) which could activate ACE2 receptors especially in organs where spike protein is more concentrated [144]. As a result, the vaccine may cause the same symptoms as COVID-19 but also potentially promote all inflammatory diseases in the medium and long term (cardiovascular, neurological, cancer, autoimmune).
This will pose a particular threat to those who already have an inflammatory disease (e.g. diabetes) or a family history of diabetes or inflammatory and autoimmune diseases [145]. Importantly, inflammation is always associated with oxidative stress in tissues. The crucial importance of oxidative stress in the pathology of COVID-19 has been largely underestimated and should be the subject of additional research efforts to identify antioxidant/anti-inflammatory treatments [146, 147]. Oxidative stress biomarkers predicted the severity and admission to intensive care of patients. Spike triggers inflammation and associated oxidative stress in COVID-19 through the biochemical pathways described in this article [148]. Moreover, it is known that oxidative stress, telomere shortening, cellular senescence, and body aging are associated [149, 150]. In COVID-19, telomere length in peripheral blood lymphocytes from patients aged 29 to 85 years has been found to be decreased in association with increased disease severity [151]. This could be linked with spike-induced aging as shown in study which measured the biological age of 117 COVID-19 survivors and 144 uninfected volunteers. The authors noted a significant increase in the biological age of patients who had COVID-19 of 10.45 years (+/- 7.29 years or 5.25 years beyond the norm) compared to 3.68 years (+/- 8.17 years) in uninfected people [152].
This phenomenon may occur both after COVID-19 infection when not treated, in long COVID, as well after vaccine in particular after various injections [153]. The toxicity of spike protein in vaccines needs to be studied and be better assessed. It is unclear if vaccines had a role to stop the pandemic and it cannot be ruled out that vaccine trials may have had a contribution in emerging variants of concern (VOC) (UK, Brazil, South Africa, India) escaping vaccine immunity and in emerging variants of interest [154]. A lot of scientists have asked for more information on vaccine safety, especially for children, given the large percentage of people who have been vaccinated. Also, it should be noted that preliminary studies on vaccines, such as AstraZeneca’s for example, were on fairly young subjects and excluded participants with severe and/or uncontrolled cardiovascular, gastrointestinal, hepatic, renal, endocrine/metabolic, neurological diseases, as well as immunocompromised people and pregnant women [155]. This likely led to an underestimation of the serious side effects among the most vulnerable populations.
In conclusion, more research is needed on spike toxicity from SARS-CoV-2 but also by the spike protein produced by mRNA and DNA vaccines. Also more information is needed on the safety of LNPs, mRNA and adjuvants of COVID-19 vaccines which may contribute to the serious side effects observed after vaccination, including thrombosis. Lethality (case-fatality ratio) for COVID-19 is on average between 0.5 and 1% compared to 0.1% for the flu worldwide [156]. Urgent studies are needed to understand the benefits and risks of this vaccination, in particular among young people who are affected very minimally by the disease, and regarding the fact that vaccination did not prevent from contamination. This is still true for COVID-19 pandemic as well as for future mRNA vaccines, either mRNA vaccines for future pandemics, or mRNA vaccines for “classical” or “usual” vaccine preventable infectious diseases, especially those developed for children. These developments should be anticipated and discussed with more clarity, through a scientific and ethical dialogue. This could encourage many citizens, whether enlightened or not, to get vaccinated, otherwise there is an induced risk of a significant decrease in confidence in scientific and medical research and treatments [157].
Our attempt, in this review, was to highlight the main immuno-inflammatory pathways as well as the key role of oxidative stress in COVID-19. This is of central importance, especially after 3 years of pandemic, in order to propose better treatments that address precisely these immuno-inflammatory pathways. We think that this has not been done yet and that it could lead to better results than what has been observed with dexamethasone for example. Furthermore, the toxicity of vaccine-induced spike protein has been neglected and underestimated since the beginning of the biggest mass vaccination in History, especially regarding what is already known on the high toxicity of spike protein. We have proposed various biochemical and physiological hypotheses in order to explain the severe side effects observed using mRNA and DNA injection technologies, often common with the severe forms of COVID-19 pathology and long COVID. This topic should be closely and carefully watched in the following years, especially on the incidence of inflammatory pathologies (cardiovascular, neurodegenerative diseases and cancers in particular) and aging.
Funding
The authors declare that this study received funding from Association BonsSens.org to cover the publication fees.
Acknowledgments
The authors thank Erin E. Moore, MA, for her work on the figures.
Conflicts of interest
All authors declare that they have no conflicts of interest.
References
- Wrapp D, Wang N, Corbett KS, Goldsmith JA, Hsieh CL, Abiona O, et al. Cryo-EM structure of the 2019-nCoV spike in the prefusion conformation. Science 367 (2020): 1260-1263.
- Lukassen S, Chua RL, Trefzer T, Kahn NC, Schneider MA, Muley T, et al. SARS-CoV-2 receptor ACE2 and TMPRSS2 are primarily expressed in bronchial transient secretory cells. EMBO J 39 (2020): e105114.
- Ogata AF, Cheng CA, Desjardins M, Senussi Y, Sherman AC, Powell M, et al. Circulating Severe Acute Respiratory Syndrome Coronavirus 2 (SARS-CoV-2) Vaccine Antigen Detected in the Plasma of mRNA-1273 Vaccine Recipients. Clin Infect Dis 74 (2022): 715-718.
- Röltgen K, Nielsen SCA, Silva O, Younes SF, Zaslavsky M, Costales C, et al. Immune imprinting, breadth of variant recognition, and germinal center response in human SARS-CoV-2 infection and vaccination. Cell 185 (2022): 1025-1040.
- Trypsteen W, Van Cleemput J, Snippenberg WV, Gerlo S, Vandekerckhove L. On the whereabouts of SARS-CoV-2 in the human body: A systematic review. PLoS Pathog 16 (2020): e1009037.
- Argenziano MG, Bruce SL, Slater CL, Tiao JR, Baldwin MR, Barr RG, et al. Characterization and clinical course of 1000 patients with coronavirus disease 2019 in New York: retrospective case series. BMJ 369 (2020): m1996.
- Huang C, Wang Y, Li X, Ren L, Zhao J, Hu Y, et al. Clinical features of patients infected with 2019 novel coronavirus in Wuhan, China. Lancet 395 (2020): 497-506.
- Lin L, Jiang X, Zhang Z, Huang S, Zhang Z, Fang Z, et al. Gastrointestinal symptoms of 95 cases with SARS-CoV-2 infection. Gut 69 (2020): 997-1001.
- Chu KH, Tsang WK, Tang CS, Lam MF, Lai FM, To KF, et al. Acute renal impairment in coronavirus-associated severe acute respiratory syndrome. Kidney Int 67 (2005): 698-705.
- Mao L, Jin H, Wang M, Hu Y, Chen S, He Q, et al. Neurologic Manifestations of Hospitalized Patients with Coronavirus Disease 2019 in Wuhan, China. JAMA Neurol 77 (2020): 683-690.
- Angeli F, Spanevello A, Reboldi G, Visca D, Verdecchia P. SARS-CoV-2 vaccines: Lights and shadows. Eur J Intern Med 88 (2021): 1-8.
- Zhang S, Liu Y, Wang X, Yang L, Li H, Wang Y, et al. SARS-CoV-2 binds platelet ACE2 to enhance thrombosis in COVID-19. J Hematol Oncol 13 (2020): 120.
- Nuovo GJ, Magro C, Shaffer T, Awad H, Suster D, Mikhail S, et al. Endothelial cell damage is the central part of COVID-19 and a mouse model induced by injection of the S1 subunit of the spike protein. Ann Diagn Pathol 51 (2021): 151682.
- Pacurari M, Kafoury R, Tchounwou PB, Ndebele K. The Renin-Angiotensin-aldosterone system in vascular inflammation and remodeling. Int J Inflam (2014): 689360.
- Crowley SD, Rudemiller NP. Immunologic Effects of the Renin-Angiotensin System. J Am Soc Nephrol 28 (2017): 1350-1361.
- Jia H. Pulmonary Angiotensin-Converting Enzyme 2 (ACE2) and Inflammatory Lung Disease. Shock 46 (2016): 239-248.
- Okamoto H, Ichikawa N. The pivotal role of the angiotensin-II-NF-κB axis in the development of COVID-19 pathophysiology. Hypertens Res 44 (2021): 126-128.
- Jamaluddin M, Meng T, Sun J, Boldogh I, Han Y, Brasier AR. Angiotensin II induces nuclear factor (NF)-kappaB1 isoforms to bind the angiotensinogen gene acute-phase response element: a stimulus-specific pathway for NF-kappaB activation. Mol Endocrinol 14 (2000): 99-113.
- Ruiz-Ortega M, Lorenzo O, Suzuki Y, Rupérez M, Egido J. Proinflammatory actions of angiotensins. Curr Opin Nephrol Hypertens 10 (2001): 321-329.
- Medina-Enríquez MM, Lopez-León S, Carlos-Escalante JA, Aponte-Torres Z, Cuapio A, Wegman-Ostrosky T. ACE2: the molecular doorway to SARS-CoV-2. Cell Biosci 10 (2020): 148.
- Peiró C, Moncada S. Substituting Angiotensin-(1-7) to Prevent Lung Damage in SARS-CoV-2 Infection. Circulation 141 (2020): 1665-1666.
- Verdecchia P, Cavallini C, Spanevello A, Angeli F. The pivotal link between ACE2 deficiency and SARS-CoV-2 infection. Eur J Intern Med 76 (2020): 14-20.
- Mahmudpour M, Roozbeh J, Keshavarz M, Farrokhi S, Nabipour I. COVID-19 cytokine storm: The anger of inflammation. Cytokine 133 (2020): 155151.
- Sodhi CP, Wohlford-Lenane C, Yamaguchi Y, Prindle T, Fulton WB, Wang S, et al. Attenuation of pulmonary ACE2 activity impairs inactivation of des-Arg9 bradykinin/BKB1R axis and facilitates LPS-induced neutrophil infiltration. Am J Physiol Lung Cell Mol Physiol 314 (2018): L17-L31.
- Tolouian R, Vahed S, Ghiyasvand S, Tolouian A, Ardalan M. COVID-19 interactions with angiotension-converting enzyme 2 and the kinin system looking at a potential treatment. J. Inj. Prev 9 (2020): e19.
- van de Veerdonk FL, Netea MG, van Deuren M, van der Meer JW, de Mast Q, Brüggemann RJ, et al. Kallikrein-kinin blockade in patients with COVID-19 to prevent acute respiratory distress syndrome. Elife 9 (2020): e57555.
- Goldin CJ, Vázquez R, Polack FP, Alvarez-Paggi D. Identifying pathophysiological bases of disease in COVID-19. Transl Med Commun 5 (2020): 15.
- Haybar H, Maniati M, Saki N, Zayeri ZD. COVID-19: imbalance of multiple systems during infection and importance of therapeutic choice and dosing of cardiac and anti-coagulant therapies. Mol Biol Rep 48 (2021): 2917-2928.
- Mansour E, Bueno FF, de Lima-Júnior JC, Palma A, Monfort-Pires M, Bombassaro B, Araujo EP, et al. Evaluation of the efficacy and safety of icatibant and C1 esterase/kallikrein inhibitor in severe COVID-19: study protocol for a three-armed randomized controlled trial. Trials 22 (2021): 71.
- Gao T, Zhu L, Liu H, Zhang X, Wang T, Fu Y, et al. Highly pathogenic coronavirus N protein aggravates inflammation by MASP-2-mediated lectin complement pathway overactivation. Signal Transduct Target Ther 7 (2022): 318.
- Gralinski LE, Sheahan TP, Morrison TE, Menachery VD, Jensen K, Leist SR, et al. Complement Activation Contributes to Severe Acute Respiratory Syndrome Coronavirus Pathogenesis. mBio 9 (2018): e01753-18.
- Jiang Y, Zhao G, Song N, Li P, Chen Y, Guo Y, et al. Blockade of the C5a-C5aR axis alleviates lung damage in hDPP4-transgenic mice infected with MERS-CoV. Emerg Microbes Infect 7 (2018): 77.
- Bumiller-Bini V, de Freitas Oliveira-Toré C, Carvalho TM, Kretzschmar GC, Gonçalves LB, Alencar NM, et al. MASPs at the crossroad between the complement and the coagulation cascades - the case for COVID-19. Genet Mol Biol 44 (2021): e20200199.
- Shajahan A, Supekar NT, Gleinich AS, Azadi P. Deducing the N- and O-glycosylation profile of the spike protein of novel coronavirus SARS-CoV-2. Glycobiology 30 (2020): 981-988.
- Zhou Y, Lu K, Pfefferle S, Bertram S, Glowacka I, Drosten C, et al. A single asparagine-linked glycosylation site of the severe acute respiratory syndrome coronavirus spike glycoprotein facilitates inhibition by mannose-binding lectin through multiple mechanisms. J Virol 84 (2010): 8753-8764.
- Ali YM, Ferrari M, Lynch NJ, Yaseen S, Dudler T, Gragerov S, et al. Lectin Pathway Mediates Complement Activation by SARS-CoV-2 Proteins. Front Immunol 12 (2021): 714511.
- Thépaut M, Luczkowiak J, Vivès C, Labiod N, Bally I, Lasala F, et al. DC/L-SIGN recognition of spike glycoprotein promotes SARS-CoV-2 trans-infection and can be inhibited by a glycomimetic antagonist. PLoS Pathog 17 (2021): e1009576.
- Gao C, Zeng J, Jia N, Stavenhagen K, Matsumoto Y, Zhang H, et al. SARS-CoV-2 Spike Protein Interacts with Multiple Innate Immune Receptors. bioRxiv (2020).
- Spear GT, Hart M, Olinger GG, Hashemi FB, Saifuddin M. The role of the complement system in virus infections. Curr Top Microbiol Immunol 260 (2001): 229-245.
- Viedt C, Hänsch GM, Brandes RP, Kübler W, Kreuzer J. The terminal complement complex C5b-9 stimulates interleukin-6 production in human smooth muscle cells through activation of transcription factors NF-kappa B and AP-1. FASEB J 14 (2000): 2370-2372.
- Ackermann M, Verleden SE, Kuehnel M, Haverich A, Welte T, Laenger F, et al. Pulmonary Vascular Endothelialitis, Thrombosis, and Angiogenesis in Covid-19. N Engl J Med 383 (2020): 120-128.
- Magro C, Mulvey JJ, Berlin D, Nuovo G, Salvatore S, Harp J, et al. Complement associated microvascular injury and thrombosis in the pathogenesis of severe COVID-19 infection: A report of five cases. Transl Res 220 (2020): 1-13.
- Llitjos JF, Leclerc M, Chochois C, Monsallier JM, Ramakers M, Auvray M, et al. High incidence of venous thromboembolic events in anticoagulated severe COVID-19 patients. J Thromb Haemost 18 (2020): 1743-1746.
- Eriksson O, Hultström M, Persson B, Lipcsey M, Ekdahl KN, Nilsson B, et al. Mannose-Binding Lectin is Associated with Thrombosis and Coagulopathy in Critically Ill COVID-19 Patients. Thromb Haemost 120 (2020): 1720-1724.
- Kenawy HI, Boral I, Bevington A. Complement-Coagulation Cross-Talk: A Potential Mediator of the Physiological Activation of Complement by Low pH. Front Immunol 6 (2015): 215.
- Berlin DA, Gulick RM, Martinez FJ. Severe Covid-19. N Engl J Med 383 (2020): 2451-2460.
- Jodele S, Köhl J. Tackling COVID-19 infection through complement-targeted immunotherapy. Br J Pharmacol 178 (2021): 2832-2848.
- Autret-Leca E, Bensouda-Grimaldi L, Jonville-Béra AP, Beau-Salinas F. [Pharmacovigilance of vaccines]. Arch Pediatr 13 (2006): 175-180.
- Lei Y, Zhang J, Schiavon CR, He M, Chen L, Shen H, et al. SARS-CoV-2 Spike Protein Impairs Endothelial Function via Downregulation of ACE 2. Circ Res 128 (2021): 1323-1326.
- Buzhdygan TP, DeOre BJ, Baldwin-Leclair A, Bullock TA, McGary HM, Khan JA, et al. The SARS-CoV-2 spike protein alters barrier function in 2D static and 3D microfluidic in-vitro models of the human blood-brain barrier. Neurobiol Dis 146 (2020): 105131.
- Hermans C, Goldman M. Thromboses et vaccins: un nouveau défi de la pandémie COVID-19. Louvain Med 140 (2021): 207-215
- Greinacher A, Thiele T, Warkentin TE, Weisser K, Kyrle PA, Eichinger S. Thrombotic Thrombocytopenia after ChAdOx1 nCov-19 Vaccination. N Engl J Med 384 (2021): 2092-2101.
- Diaz GA, Parsons GT, Gering SK, Meier AR, Hutchinson IV, Robicsek A. Myocarditis and Pericarditis After Vaccination for COVID-19. JAMA 326 (2021): 1210-1212.
- Dionne A, Sperotto F, Chamberlain S, Baker AL, Powell AJ, Prakash A, et al. Association of Myocarditis with BNT162b2 Messenger RNA COVID-19 Vaccine in a Case Series of Children. JAMA Cardiol 6 (2021): 1446-1450.
- Seneff S, Nigh G. Worse Than the Disease? Reviewing Some Possible Unintended Consequences of the mRNA Vaccines Against COVID-19. International Journal of Vaccine Theory, Practice, and Research 2 (2021): 38–79.
- Bernardi S, Toffoli B, Zennaro C, Tikellis C, Monticone S, Losurdo P, et al. High-salt diet increases glomerular ACE/ACE2 ratio leading to oxidative stress and kidney damage. Nephrol Dial Transplant 27 (2012): 1793-1800.
- Lavrentyev EN, Malik KU. High glucose-induced Nox1-derived superoxides downregulate PKC-betaII, which subsequently decreases ACE2 expression and ANG (1-7) formation in rat VSMCs. Am J Physiol Heart Circ Physiol 296 (2009): H106-18.
- Mizuiri S, Hemmi H, Arita M, Ohashi Y, Tanaka Y, Miyagi M, et al. Expression of ACE and ACE2 in individuals with diabetic kidney disease and healthy controls. Am J Kidney Dis 51 (2008): 613-623.
- Yuan YM, Luo L, Guo Z, Yang M, Ye RS, Luo C. Activation of renin-angiotensin-aldosterone system (RAAS) in the lung of smoking-induced pulmonary arterial hypertension (PAH) rats. J Renin Angiotensin Aldosterone Syst 16 (2015): 249-253.
- Kehoe PG, Wong S, Al Mulhim N, Palmer LE, Miners JS. Angiotensin-converting enzyme 2 is reduced in Alzheimer's disease in association with increasing amyloid-β and tau pathology. Alzheimers Res Ther 8 (2016): 50.
- Schneider J, Pease D, Navaratnarajah C, Halfmann P, Clemens D, Ye D, et al. SARS-CoV-2 direct cardiac damage through spike-mediated cardiomyocyte fusion. Research Square (2020).
- Lazebnik Y. Cell fusion as a link between the SARS-CoV-2 spike protein, COVID-19 complications, and vaccine side effects. Oncotarget 12 (2021): 2476-2488.
- Wenzhong L, Hualan L. COVID-19: the CaMKII-like system of S protein drives membrane fusion and induces syncytial multinucleated giant cells. Immunol Res 69 (2021): 496-519.
- Navaratnarajah CK, Pease DR, Halfmann PJ, Taye B, Barkhymer A, Howell KG, et al. Highly Efficient SARS-CoV-2 Infection of Human Cardiomyocytes: Spike Protein-Mediated Cell Fusion and Its Inhibition. J Virol 95 (2021): e0136821.
- Clemens DJ, Ye D, Zhou W, Kim CSJ, Pease DR, Navaratnarajah CK, et al. SARS-CoV-2 spike protein-mediated cardiomyocyte fusion may contribute to increased arrhythmic risk in COVID-19. PLoS One 18 (2023): e0282151.
- Sfera A. COVID-19 mRNA Vaccines and Pathological Cell-Cell Fusion: An Unintended Consequence. J Immunol Res Infect Dis 2 (2022): 2771-4691.
- Sinha N, Thakur AK. Likelihood of amyloid formation in COVID-19-induced ARDS. Trends Microbiol 29 (2021): 967-969.
- Yamakawa M, Lynch S, Townley RA. Case of cerebral amyloid angiopathy related inflammation after vaccination against SARS-CoV-2. Neuroimmunology Reports 2 (2022): 100120-100120.
- Nyström S, Hammarström P. Amyloidogenesis of SARS-CoV-2 Spike Protein. J Am Chem Soc 144 (2022): 8945-8950.
- Gonçalves de Andrade E, Šimoncicová E, Carrier M, Vecchiarelli HA, Robert MÈ, Tremblay MÈ. Microglia Fighting for Neurological and Mental Health: On the Central Nervous System Frontline of COVID-19 Pandemic. Front Cell Neurosci 15 (2021): 647378.
- Maiese A, Manetti AC, Bosetti C, Del Duca F, La Russa R, Frati P, et al. SARS-CoV-2 and the brain: A review of the current knowledge on neuropathology in COVID-19. Brain Pathol 31 (2021): e13013.
- Matschke J, Lütgehetmann M, Hagel C, Sperhake JP, Schröder AS, Edler C, et al. Neuropathology of patients with COVID-19 in Germany: a post-mortem case series. Lancet Neurol 19 (2020): 919-929.
- Mao L, Jin H, Wang M, Hu Y, Chen S, He Q, et al. Neurologic Manifestations of Hospitalized Patients with Coronavirus Disease 2019 in Wuhan, China. JAMA Neurol 77 (2020): 683-690.
- Baig AM, Khaleeq A, Ali U, Syeda H. Evidence of the COVID-19 Virus Targeting the CNS: Tissue Distribution, Host-Virus Interaction, and Proposed Neurotropic Mechanisms. ACS Chem Neurosci 11 (2020): 995-998.
- Chen R, Wang K, Yu J, Howard D, French L, Chen Z, et al. The Spatial and Cell-Type Distribution of SARS-CoV-2 Receptor ACE2 in the Human and Mouse Brains. Front Neurol 11 (2020): 573095.
- Desforges M, Le Coupanec A, Dubeau P, Bourgouin A, Lajoie L, Dubé M, et al. Human Coronaviruses and Other Respiratory Viruses: Underestimated Opportunistic Pathogens of the Central Nervous System. Viruses 12 (2019): 14.
- Pellegrini L, Albecka A, Mallery DL, Kellner MJ, Paul D, Carter AP, et al. SARS-CoV-2 Infects the Brain Choroid Plexus and Disrupts the Blood-CSF Barrier in Human Brain Organoids. Cell Stem Cell 27 (2020): 951-961.
- Paniz-Mondolfi A, Bryce C, Grimes Z, Gordon RE, Reidy J, Lednicky J, et al. Central nervous system involvement by severe acute respiratory syndrome coronavirus-2 (SARS-CoV-2). J Med Virol 92 (2020): 699-702.
- Schwabenland M, Salié H, Tanevski J, Killmer S, Lago MS, Schlaak AE, et al. Deep spatial profiling of human COVID-19 brains reveals neuroinflammation with distinct microanatomical microglia-T-cell interactions. Immunity 54 (2021): 1594-1610.
- Generoso JS, Barichello de Quevedo JL, Cattani M, Lodetti BF, Sousa L, Collodel A, Diaz AP, Dal-Pizzol F. Neurobiology of COVID-19: how can the virus affect the brain. Braz J Psychiatry 43 (2021): 650-664.
- Lou JJ, Movassaghi M, Gordy D, Olson MG, Zhang T, Khurana MS, et al. Neuropathology of COVID-19 (neuro-COVID): clinicopathological update. Free Neuropathol 2 (2021): 2.
- Rhea EM, Logsdon AF, Hansen KM, Williams LM, Reed MJ, Baumann KK, et al. The S1 protein of SARS-CoV-2 crosses the blood-brain barrier in mice. Nat Neurosci 24 (2021): 368-378.
- Bahl K, Senn JJ, Yuzhakov O, Bulychev A, Brito LA, Hassett KJ, et al. Preclinical and Clinical Demonstration of Immunogenicity by mRNA Vaccines against H10N8 and H7N9 Influenza Viruses. Mol Ther 25 (2017): 1316-1327.
- EMA Public Assessment Report on Pfizer-BioNTech Vaccine (2020).
- Olajide OA, Iwuanyanwu VU, Adegbola OD, Al-Hindawi AA. SARS-CoV-2 Spike Glycoprotein S1 Induces Neuroinflammation in BV-2 Microglia. Mol Neurobiol 59 (2022): 445-458.
- Pekna M, Pekny M. The Complement System: A Powerful Modulator and Effector of Astrocyte Function in the Healthy and Diseased Central Nervous System. Cells 10 (2021): 1812.
- Lian H, Litvinchuk A, Chiang AC, Aithmitti N, Jankowsky JL, Zheng H. Astrocyte-Microglia Cross Talk through Complement Activation Modulates Amyloid Pathology in Mouse Models of Alzheimer's Disease. J Neurosci 36 (2016): 577-589.
- Sarlus H, Heneka MT. Microglia in Alzheimer's disease. J Clin Invest 127 (2017): 3240-3249.
- Hong S, Beja-Glasser VF, Nfonoyim BM, Frouin A, Li S, Ramakrishnan S, et al. Complement and microglia mediate early synapse loss in Alzheimer mouse models. Science 352 (2016): 712-716.
- Yepes M. Neurological Complications of SARS-CoV-2 Infection and COVID-19 Vaccines: From Molecular Mechanisms to Clinical Manifestations. Curr Drug Targets 23 (2022): 1620-1638.
- Li X, Cai Y, Zhang Z, Zhou J. Glial and Vascular Cell Regulation of the Blood-Brain Barrier in Diabetes. Diabetes Metab J 46 (2022): 222-238.
- Klempin F, Mosienko V, Matthes S, Villela DC, Todiras M, Penninger JM, et al. Depletion of angiotensin-converting enzyme 2 reduces brain serotonin and impairs the running-induced neurogenic response. Cell Mol Life Sci 75 (2018): 3625-3634.
- de Melo LA, Almeida-Santos AF. Neuropsychiatric Properties of the ACE2/Ang-(1-7)/Mas Pathway: A Brief Review. Protein Pept Lett 27 (2020): 476-483.
- Konstantinidis I, Tsakiropoulou E, Hähner A, de With K, Poulas K, Hummel T. Olfactory dysfunction after coronavirus disease 2019 (COVID-19) vaccination. Int Forum Allergy Rhinol 11 (2021): 1399-1401.
- Theoharides TC. Could SARS-CoV-2 Spike Protein Be Responsible for Long-COVID Syndrome. Mol Neurobiol 59 (2022): 1850-1861.
- Hassnine AA, Elsayed AM. COVID-19 in Cirrhotic Patients: Is Portal Vein Thrombosis a Potential Complication. Can J Gastroenterol Hepatol (2022): 5900468.
- Melas N. Portal vein thrombosis occurring after the first dose of mRNA SARS-CoV-2 vaccine in a patient with antiphospholipid syndrome. Thrombosis Update 5 (2021): 100069.
- Öcal O, Stecher SS, Wildgruber M. Portal vein thrombosis associated with ChAdOx1 nCov-19 vaccination. Lancet Gastroenterol Hepatol 6 (2021): 676.
- Repp ML, Cohen S, Kibbey C. Acute Portal Vein Thrombosis Secondary to COVID-19 Vaccination. Cureus 14 (2022): e26825.
- Bril F, Al Diffalha S, Dean M, Fettig DM. Autoimmune hepatitis developing after coronavirus disease 2019 (COVID-19) vaccine: Causality or casualty. J Hepatol 75 (2021): 222-224.
- Rocco A, Sgamato C, Compare D, Nardone G. Autoimmune hepatitis following SARS-CoV-2 vaccine: May not be a casuality. J Hepatol 75 (2021): 728-729.
- Rela M, Jothimani D, Vij M, Rajakumar A, Rammohan A. Auto-immune hepatitis following COVID vaccination. J Autoimmun 123 (2021): 102688.
- Lee WS, Wheatley AK, Kent SJ, DeKosky BJ. Antibody-dependent enhancement and SARS-CoV-2 vaccines and therapies. Nat Microbiol 5 (2020): 1185-1191.
- Zhang B, Yu X, Liu J, Liu J, Liu P. COVID-19 vaccine and menstrual conditions in female: data analysis of the Vaccine Adverse Event Reporting System (VAERS). BMC Womens Health 22 (2022): 403.
- Muhaidat N, Alshrouf MA, Azzam MI, Karam AM, Al-Nazer MW, Al-Ani A. Menstrual Symptoms After COVID-19 Vaccine: A Cross-Sectional Investigation in the MENA Region. Int J Womens Health 14 (2022): 395-404.
- Laganà AS, Veronesi G, Ghezzi F, Ferrario MM, Cromi A, Bizzarri M, et al. Evaluation of menstrual irregularities after COVID-19 vaccination: Results of the MECOVAC survey. Open Med (Wars) 17 (2022): 475-484.
- Nazir M, Asghar S, Rathore MA, Shahzad A, Shahid A, Ashraf Khan A, et al. Menstrual abnormalities after COVID-19 vaccines: A systematic review. Vacunas 23 (2022): S77-S87.
- Farland LV, Khan SM, Shilen A, Heslin KM, Ishimwe P, Allen AM, et al. COVID-19 vaccination and changes in the menstrual cycle among vaccinated persons. Fertil Steril 119 (2023): 392-400.
- Lessans N, Rottenstreich A, Stern S, Gilan A, Saar TD, Porat S, et al. The effect of BNT162b2 SARS-CoV-2 mRNA vaccine on menstrual cycle symptoms in healthy women. Int J Gynaecol Obstet 160 (2023): 313-318.
- Gilan A, Laster-Haim S, Rottenstreich A, Porat S, Lessans N, Saar TD, et al. The effect of SARS-CoV-2 BNT162b2 vaccine on the symptoms of women with endometriosis. Arch Gynecol Obstet 307 (2023): 121-127.
- Edelman A, Boniface ER, Benhar E, Han L, Matteson KA, Favaro C, et al. Association Between Menstrual Cycle Length and Coronavirus Disease 2019 (COVID-19) Vaccination: A U.S. Cohort. Obstet Gynecol 139 (2022): 481-489.
- Saadedine M, El Sabeh M, Borahay MA, Daoud G. The influence of COVID-19 infection-associated immune response on the female reproductive system†. Biol Reprod 108 (2023): 172-182.
- Jing Y, Run-Qian L, Hao-Ran W, Hao-Ran C, Ya-Bin L, Yang G, et al. Potential influence of COVID-19/ACE2 on the female reproductive system. Mol Hum Reprod 26 (2020): 367-373.
- Mendoza M, Garcia-Ruiz I, Maiz N, Rodo C, Garcia-Manau P, Serrano B, et al. Pre-eclampsia-like syndrome induced by severe COVID-19: a prospective observational study. BJOG 127 (2020): 1374-1380.
- Girardi G, Lingo JJ, Fleming SD, Regal JF. Essential Role of Complement in Pregnancy: From Implantation to Parturition and Beyond. Front Immunol 11 (2020): 1681.
- Zauche LH, Wallace B, Smoots AN, Olson CK, Oduyebo T, Kim SY, et al. Receipt of mRNA Covid-19 Vaccines and Risk of Spontaneous Abortion. N Engl J Med 385 (2021): 1533-1535.
- Beltramello M, Williams KL, Simmons CP, Macagno A, Simonelli L, Quyen NT, et al. The human immune response to Dengue virus is dominated by highly cross-reactive antibodies endowed with neutralizing and enhancing activity. Cell Host Microbe 8 (2010): 271-283.
- Bardina SV, Bunduc P, Tripathi S, Duehr J, Frere JJ, Brown JA, et al. Enhancement of Zika virus pathogenesis by preexisting antiflavivirus immunity. Science 356 (2017): 175-180.
- Takada A, Feldmann H, Ksiazek TG, Kawaoka Y. Antibody-dependent enhancement of Ebola virus infection. J Virol 77 (2003): 7539-7544.
- Huisman W, Martina BE, Rimmelzwaan GF, Gruters RA, Osterhaus AD. Vaccine-induced enhancement of viral infections. Vaccine 27 (2009): 505-512.
- Wang SF, Tseng SP, Yen CH, Yang JY, Tsao CH, Shen CW, et al. Antibody-dependent SARS coronavirus infection is mediated by antibodies against spike proteins. Biochem Biophys Res Commun 451 (2014): 208-214.
- Du L, Tai W, Zhou Y, Jiang S. Vaccines for the prevention against the threat of MERS-CoV. Expert Rev Vaccines 15 (2016): 1123-1134.
- Vennema H, de Groot RJ, Harbour DA, Dalderup M, Gruffydd-Jones T, Horzinek MC, et al. Early death after feline infectious peritonitis virus challenge due to recombinant vaccinia virus immunization. J Virol 64 (1990): 1407-1409.
- Yahi N, Chahinian H, Fantini J. Infection-enhancing anti-SARS-CoV-2 antibodies recognize both the original Wuhan/D614G strain and Delta variants. A potential risk for mass vaccination. J Infect 83 (2021): 607-635.
- Guérin P, Yahi N, Azzaz F, Chahinian H, Sabatier JM, Fantini J. Structural Dynamics of the SARS-CoV-2 Spike Protein: A 2-Year Retrospective Analysis of SARS- CoV-2 Variants (from Alpha to Omicron) Reveals an Early Divergence between Conserved and Variable Epitopes. Molecules 27 (2022): 3851.
- Ulrich H, Pillat MM, Tárnok A. Dengue Fever, COVID-19 (SARS-CoV-2), and Antibody-Dependent Enhancement (ADE): A Perspective. Cytometry A 97 (2020): 662-667.
- Cardozo T, Veazey R. Informed consent disclosure to vaccine trial subjects of risk of COVID-19 vaccines worsening clinical disease. Int J Clin Pract 75 (2021): e13795.
- Sridhar P, Singh A, Salomon N, Steiger D. Vaccine-induced antibody dependent enhancement in Covid-19. Chest 162 (2022): A646–7.
- Bando T, Takei R, Mutoh Y, Sasano H, Yamano Y, Yokoyama T, et al. Two cases of acute respiratory failure following SARS-CoV-2 vaccination in post-COVID-19 pneumonia. Respirol Case Rep 10 (2022): e0995.
- Hirschbühl K, Schaller T, Märkl B, Claus R, Sipos E, Rentschler L, et al. High viral loads: what drives fatal cases of COVID-19 in vaccinees? - an autopsy study. Mod Pathol 35 (2022): 1013-1021.
- Doulberis M, Papaefthymiou A, Kotronis G, Gialamprinou D, Soteriades ES, Kyriakopoulos A, et al. Does COVID-19 Vaccination Warrant the Classical Principle "ofelein i mi vlaptin". Medicina (Kaunas) 57 (2021): 253.
- Halma MTJ, Rose J, Lawrie T. The Novelty of mRNA Viral Vaccines and Potential Harms: A Scoping Review. J 6 (2023): 220-235.
- Talotta R. Do COVID-19 RNA-based vaccines put at risk of immune-mediated diseases? In reply to "potential antigenic cross-reactivity between SARS-CoV-2 and human tissue with a possible link to an increase in autoimmune diseases". Clin Immunol 224 (2021): 108665.
- Mouliou DS, Dardiotis E. Current Evidence in SARS-CoV-2 mRNA Vaccines and Post-Vaccination Adverse Reports: Knowns and Unknowns. Diagnostics (Basel) 12 (2022): 1555.
- MODERNA, INC. WO 2017/099823 Al. COMPOSITIONS AND METHODS FOR DELIVERY OF THERAPEUTIC AGENTS (2017).
- Ndeupen S, Qin Z, Jacobsen S, Bouteau A, Estanbouli H, Igyártó BZ. The mRNA-LNP platform's lipid nanoparticle component used in preclinical vaccine studies is highly inflammatory. iScience 1724 (2021): 103479.
- Wan Y, Shang J, Graham R, Baric RS, Li F. Receptor Recognition by the Novel Coronavirus from Wuhan: an Analysis Based on Decade-Long Structural Studies of SARS Coronavirus. J Virol 94 (2020): e00127-20.
- Chen IY, Chang SC, Wu HY, Yu TC, Wei WC, Lin S, et al. Upregulation of the chemokine (C-C motif) ligand 2 via a severe acute respiratory syndrome coronavirus spike-ACE2 signaling pathway. J Virol 84 (2010): 7703-7712.
- Kuba K, Imai Y, Rao S, Gao H, Guo F, Guan B, et al. A crucial role of angiotensin converting enzyme 2 (ACE2) in SARS coronavirus-induced lung injury. Nat Med 11 (2005): 875-879.
- Patra T, Meyer K, Geerling L, Isbell TS, Hoft DF, Brien J, et al. SARS-CoV-2 spike protein promotes IL-6 trans-signaling by activation of angiotensin II receptor signaling in epithelial cells. PLoS Pathog 16 (2020): e1009128.
- Weingartl H, Czub M, Czub S, Neufeld J, Marszal P, Gren J, et al. Immunization with modified vaccinia virus Ankara-based recombinant vaccine against severe acute respiratory syndrome is associated with enhanced hepatitis in ferrets. J Virol 78 (2004): 12672-12676.
- Czub M, Weingartl H, Czub S, He R, Cao J. Evaluation of modified vaccinia virus Ankara based recombinant SARS vaccine in ferrets. Vaccine 23 (2005): 2273-2279.
- Jaume M, Yip MS, Kam YW, Cheung CY, Kien F, Roberts A, et al. SARS CoV subunit vaccine: antibody-mediated neutralisation and enhancement. Hong Kong Med J 18 (2012): 31-36.
- Cosentino M, Marino F. The spike hypothesis in vaccine-induced adverse effects: questions and answers. Trends Mol Med 28 (2022): 797-799.
- Yano M, Morioka T, Natsuki Y, Sasaki K, Kakutani Y, Ochi A, et al. New-onset Type 1 Diabetes after COVID-19 mRNA Vaccination. Intern Med 61 (2022): 1197-1200.
- Cecchini R, Cecchini AL. SARS-CoV-2 infection pathogenesis is related to oxidative stress as a response to aggression. Med Hypotheses 143 (2020): 110102.
- Delgado-Roche, L, Mesta, F. Oxidative Stress as Key Player in Severe Acute Respiratory Syndrome Coronavirus (SARS-CoV) Infection. Arch Med Res 51 (2020): 384-387.
- Ducastel M, Chenevier-Gobeaux C, Ballaa Y, Meritet JF, Brack M, Chapuis N, et al. Oxidative Stress and Inflammatory Biomarkers for the Prediction of Severity and ICU Admission in Unselected Patients Hospitalized with COVID-19. Int J Mol Sci 22 (2021): 7462.
- Richter T, von Zglinicki T. A continuous correlation between oxidative stress and telomere shortening in fibroblasts. Exp Gerontol 42 (2007): 1039-1042.
- Kawanishi S, Oikawa S. Mechanism of telomere shortening by oxidative stress. Ann N Y Acad Sci 1019 (2004): 278-284.
- Sanchez-Vazquez R, Guío-Carrión A, Zapatero-Gaviria A, Martínez P, Blasco MA. Shorter telomere lengths in patients with severe COVID-19 disease. Aging (Albany NY) 13 (2021): 1-15.
- Mongelli A, Barbi V, Gottardi Zamperla M, Atlante S, Forleo L, Nesta M, et al. Evidence for Biological Age Acceleration and Telomere Shortening in COVID-19 Survivors. Int J Mol Sci 22 (2021): 6151.
- Azalbert X, Bouthors AT, Brack M, Cerdan D, Chesnut W, Guillaume G, et al. FranceSoir (2021).
- Tan M, Lounnas V, Azalbert X, Perronne C. May vaccines select SARS-CoV-2 variants more readily escaping immunity - an analysis of public data. Archives of Microbiology & Immunology 5 (2021): 316-324.
- Voysey M, Clemens SAC, Madhi SA, Weckx LY, Folegatti PM, Aley PK. et al. Safety and efficacy of the ChAdOx1 nCoV-19 vaccine (AZD1222) against SARS-CoV-2: an interim analysis of four randomised controlled trials in Brazil, South Africa, and the UK. Lancet 397 (2021): 99-111.
- JHU Data. https://coronavirus.jhu.edu/data/mortality
- Deruelle F. The pharmaceutical industry is dangerous to health. Further proof with COVID-19. Surg Neurol Int 13 (2022): 475.