Role of Ribonucleotides in Improving Muscle Cell Function
Article Information
Francesca Uberti*#, Sara Ruga#, Vera Morsanuto, Rebecca Galla, Mahitab Farghali, Claudio Molinari
Laboratory Physiology, Department of Translational Medicine, Novara, Italy
*Corresponding Author: Francesca Uberti, Laboratory Physiology, Department of Translational Medicine, via Solaroli 17, 28100 Novara, Italy
Received: 14 September 2020; Accepted: 26 September 2020; Published: 20 October 2020
Citation:
Francesca Uberti, Sara Ruga, Vera Morsanuto, Rebecca Galla, Mahitab Farghali, Claudio Molinari. Role of Ribonucleotides in Improving Muscle Cell Function. Journal of Food Science and Nutrition Research 3 (2020): 231-251.
View / Download Pdf Share at FacebookAbstract
Based on data present in literature about some beneficial effects of nucleotides in reducing inflammation, and on lacking information about the mechanisms underlying the cellular aspects of exercise, the aim of this study was to examine the effects of the administration of a mixture of nucleotides and nucleotide-ribose on energy metabolism and contractility in cultured skeletal muscle cells in presence or absence of caffeine-dependent hyper-contraction. C2C12 cells were treated with various nucleotides forms, named Ribodiet®, RIBOCARE® MIX 1, RIBOCARE® MIX 2, and RNA-Na. Cell viability, calcium, and magnesium movements and the main kinases involved in glucose uptake have been analyzed. The level of glycogen and the presence of lactate have been evaluated as well. Since some important findings were obtained, additional experiments analyzed the effects of the same nucleotide forms under severe exercise conditions in order to understand the correct human use of nucleotides as food supplements to better support the exercise effects. The results obtained show for the first time, a direct effect of nucleotides on energy metabolism and calcium/ magnesium movements during in vitro exercise simulation and provide useful indications to support muscle cells during exercise or during aging.
Keywords
Nucleotides, Muscle cells, Fatigue, Exercise physiology, Caffeine-induced contraction
Nucleotides articles; Muscle cells articles; Fatigue articles; Exercise physiology articles; Caffeine-induced contraction articles
Nucleotides articles Nucleotides Research articles Nucleotides review articles Nucleotides PubMed articles Nucleotides PubMed Central articles Nucleotides 2023 articles Nucleotides 2024 articles Nucleotides Scopus articles Nucleotides impact factor journals Nucleotides Scopus journals Nucleotides PubMed journals Nucleotides medical journals Nucleotides free journals Nucleotides best journals Nucleotides top journals Nucleotides free medical journals Nucleotides famous journals Nucleotides Google Scholar indexed journals Muscle cells articles Muscle cells Research articles Muscle cells review articles Muscle cells PubMed articles Muscle cells PubMed Central articles Muscle cells 2023 articles Muscle cells 2024 articles Muscle cells Scopus articles Muscle cells impact factor journals Muscle cells Scopus journals Muscle cells PubMed journals Muscle cells medical journals Muscle cells free journals Muscle cells best journals Muscle cells top journals Muscle cells free medical journals Muscle cells famous journals Muscle cells Google Scholar indexed journals Fatigue articles Fatigue Research articles Fatigue review articles Fatigue PubMed articles Fatigue PubMed Central articles Fatigue 2023 articles Fatigue 2024 articles Fatigue Scopus articles Fatigue impact factor journals Fatigue Scopus journals Fatigue PubMed journals Fatigue medical journals Fatigue free journals Fatigue best journals Fatigue top journals Fatigue free medical journals Fatigue famous journals Fatigue Google Scholar indexed journals Exercise physiology articles Exercise physiology Research articles Exercise physiology review articles Exercise physiology PubMed articles Exercise physiology PubMed Central articles Exercise physiology 2023 articles Exercise physiology 2024 articles Exercise physiology Scopus articles Exercise physiology impact factor journals Exercise physiology Scopus journals Exercise physiology PubMed journals Exercise physiology medical journals Exercise physiology free journals Exercise physiology best journals Exercise physiology top journals Exercise physiology free medical journals Exercise physiology famous journals Exercise physiology Google Scholar indexed journals Caffeine-induced contraction articles Caffeine-induced contraction Research articles Caffeine-induced contraction review articles Caffeine-induced contraction PubMed articles Caffeine-induced contraction PubMed Central articles Caffeine-induced contraction 2023 articles Caffeine-induced contraction 2024 articles Caffeine-induced contraction Scopus articles Caffeine-induced contraction impact factor journals Caffeine-induced contraction Scopus journals Caffeine-induced contraction PubMed journals Caffeine-induced contraction medical journals Caffeine-induced contraction free journals Caffeine-induced contraction best journals Caffeine-induced contraction top journals Caffeine-induced contraction free medical journals Caffeine-induced contraction famous journals Caffeine-induced contraction Google Scholar indexed journals aging articles aging Research articles aging review articles aging PubMed articles aging PubMed Central articles aging 2023 articles aging 2024 articles aging Scopus articles aging impact factor journals aging Scopus journals aging PubMed journals aging medical journals aging free journals aging best journals aging top journals aging free medical journals aging famous journals aging Google Scholar indexed journals antioxidant articles antioxidant Research articles antioxidant review articles antioxidant PubMed articles antioxidant PubMed Central articles antioxidant 2023 articles antioxidant 2024 articles antioxidant Scopus articles antioxidant impact factor journals antioxidant Scopus journals antioxidant PubMed journals antioxidant medical journals antioxidant free journals antioxidant best journals antioxidant top journals antioxidant free medical journals antioxidant famous journals antioxidant Google Scholar indexed journals anti-aging properties articles anti-aging properties Research articles anti-aging properties review articles anti-aging properties PubMed articles anti-aging properties PubMed Central articles anti-aging properties 2023 articles anti-aging properties 2024 articles anti-aging properties Scopus articles anti-aging properties impact factor journals anti-aging properties Scopus journals anti-aging properties PubMed journals anti-aging properties medical journals anti-aging properties free journals anti-aging properties best journals anti-aging properties top journals anti-aging properties free medical journals anti-aging properties famous journals anti-aging properties Google Scholar indexed journals cell-mediated immunity articles cell-mediated immunity Research articles cell-mediated immunity review articles cell-mediated immunity PubMed articles cell-mediated immunity PubMed Central articles cell-mediated immunity 2023 articles cell-mediated immunity 2024 articles cell-mediated immunity Scopus articles cell-mediated immunity impact factor journals cell-mediated immunity Scopus journals cell-mediated immunity PubMed journals cell-mediated immunity medical journals cell-mediated immunity free journals cell-mediated immunity best journals cell-mediated immunity top journals cell-mediated immunity free medical journals cell-mediated immunity famous journals cell-mediated immunity Google Scholar indexed journals
Article Details
1. Introduction
Fatigue is a feeling of extreme tiredness, which can result in a broad range of physical and mental unfitness [1, 2]. From the point of view of energy metabolism, it is characterized by the depletion of energy sources, the accumulation of end products, the decrease in glycemic levels, and glycogen consumption [3]. For this reason, efforts are needed to develop nutritional interventions capable of safely and effectively preventing adverse internal effects such as increased oxidative stress [4], and damage to mitochondria [5]. Interventions that reduce oxidative damage can effectively relieve fatigue, as suggested by the results of studies that have shown that the administration of antioxidant agents produced beneficial effects in mice [6, 7], also during the recovery phase after exercise [8]. In this context, the administration of nucleotides can represent a new intervention strategy, because they have demonstrated many beneficial functions, as both an ergogenic and immunostimulating additive including antitumor activity, immune modulation, protective capacity of the liver, normalization of metabolism, and improvement of exercise performance indices [9-13].
Additionally, nucleotides exhibit superior antioxidant and anti-aging properties, as demonstrated by a rat study [14].
Nucleotides are low-molecular-weight endogenously synthesized intracellular compounds, which form the basic units of the nucleic acids DNA and RNA, essential for cell division [15]. They are phosphoric nucleoside esters, made up of three components: a weakly basic nitrogenous compound, a pentose sugar, and one or more phosphate groups. The most important nucleotides are adenosine, guanosine, inosine, cytidine, and uridine monophosphates. Nucleotides are considered ‘semi-essential’ nutrients. This means that endogenous production meets the requirements in the basic maintenance condition, but exogenous administration is necessary for conditions such as childhood, stress, or tissue damage [16]. On the one hand, some tissues have a limited capacity for de novo nucleotide synthesis, and therefore require bases provided exogenously, on the other hand, some tissues regenerate more frequently than others and therefore require more nucleotides to support this activity [17]. For example, the intestinal mucosa, haematopoietic cells of the bone marrow, leucocytes, erythrocytes, and lymphocytes are not capable of de novo synthesis, and thus utilize the salvage pathway, suggesting that exogenous supply of nucleotides via the diet might be important for these cells [18]. On the other hand, individuals with a higher growth rate, such as pregnant women or children, also fall into the category of people with greater need, but these are not the only factors because also malnutrition or infections, injuries, damage to the tract intestinal (e.g. due to inflammatory bowel disease, IBD) may increase dietary needs. Indeed, nucleotide supplementation has also been linked to a reduction in IBD symptoms [19]. Moreover, nucleotides have a direct effect on the maintenance of intestinal mucosal integrity. It has been demonstrated that nucleotide supplementation in young rats increases the weight of the intestinal mucosa, villus height (by 25%), and the activity of brush border enzymes (maltase, sucrase, and lactase), suggesting an acceleration of gut growth and differentiation [20].
In addition, supplementation with a nucleoside-nucleotide mixture increases recovery after food deprivation, infection, or protein deficiency. Small intestine atrophy and decreased activity of brush border enzymes in rats quickly resolved with nucleotide supplementation [21]. As regards microbiota health status, in vivo studies show that dietary supplementation with nucleotides improves the intestinal flora and stimulates the growth of bifidobacteria [22]. Food nucleotides favor the development of the intestinal microbiota with a predominance of bifidobacteria and lactobacilli and a lower percentage of Gram-negative enterobacteria. Furthermore, nucleotides are mainly used in baby food as shown by many studies on healthy term infants where nucleotide supplementation is able to reduce the risk of diarrhea [18] or in a study about parenteral and baby milk formulas to improve intestinal health and to develop the immune system [20]. Moreover, another important field of application includes the immune system in which nucleotides have been shown to enhance capabilities, during cellular response to pathogens or viruses [23]. Nucleotides have also been shown to reduce the symptoms of common cold, including earache and sinus pain, and are especially effective when supplemented early in the infection [24].
Finally, as for immune system modulation, nucleotides influence both humoral and cell-mediated immunity: they accelerate T-cell-dependent antibody production and seem to exert actions on T-helper cells during antigen presentation, perhaps during cognitive cell-cell interactions. A nucleoside-nucleotide mixture stimulates the proliferation, differentiation, and maturation of neutrophils [20]. Nucleotides cause a transient increase in natural killer cell cytotoxicity, interleukin-2 production, and interferon-gamma secretion, and lower macrophage activation [25].
A recent application of nucleotide supplementation includes the effects on muscle cells in particular during fatigue and post-exercise recovery phase, although the intracellular mechanisms are poorly understood. Nucleotides are the building blocks for a variety of molecules, including ATP, which is broken down in the skeletal muscle cells to provide fuel for exercise. Nucleotides can be obtained from the diet and produced or retrieved by the body. Although nucleotides provide the building blocks of ATP, it has been suggested that sugar ribose limits the rate for synthesizing ATP. Previous studies have shown a decrease in ATP levels in the muscle after intense exercise, and this is linked to fatigue. Other studies have also reported benefits of nucleotide and/or ribose supplementation on exercise performance. However, no potential link between ATP metabolism and physical performance has yet been explored [26]. The main problem during exercise is to maintain adequate circulation and energy production but the main consequence includes free radical production that can lead to DNA damage or cell death. In this context the balance between oxidant and antioxidant levels is more important; if the antioxidant levels are low, the secondary effect can lead to inflammation and tissue damage. In this case, nucleotides are able to repair damaged DNA [27]. In addition, during the increase of muscle tone, nucleotides are essential for protein synthesis, and to reduce cortisol levels and increase secretory IgA [28].
The main important consequences of exercise and fatigue are skeletal muscle cramps that occur during or shortly following exercise in healthy individuals with no underlying metabolic, neurological, or endocrine pathology have been termed exercise-associated muscle cramps [29]. This condition can be present also in patients with several diseases influencing the quality of life [30]. Based on previous data on the use of ribose, the administration of nucleotide mixtures may be a possible new strategy to reduce or prevent exercise-induced muscle cramps and pain [31].
The aim of this study was to examine the effects of administering a mixture of nucleotides and nucleotide-ribose on fuel utilization and performance in skeletal muscle cells in the presence or absence of hyper-contraction caused by the presence of caffeine.
2. Material and Methods
2.1 Cell culture
Murine C2C12 myoblastic cell line was grown in Dulbecco’s Modified Eagle Medium (DMEM; Sigma-Aldrich, Milan, Italy) supplemented with 10% FBS (Sigma-Aldrich, Milan, Italy), 100 U/ml penicillin/streptomycin and maintained in an incubator at 37°C 5% CO2. The cells were maintained at 40-70% density in order to have cell growth (cell proliferation) without cell differentiation. For experiments, cells were plated under different protocols: 1x104 on 96-well plates to analyze the mitochondrial activity by MTT test; the 10 × 104 cells/well in six-well plates in order to investigate the intracellular pathways activated by western blot and the Glucose/ Glycogen and lactate productions. The cell cycle stage of the subconfluence cells was synchronized by being cultured in DMEM for 24 h. The cells were then transferred to the growth medium at 2% FBS containing various nucleotides for a range time starting from 1h to 7h and 24h to verify the early phase of differentiation and the main mechanism activated. The nucleotides were tested in the presence or absence of high concentration of caffeine (2.5 mM) in order to mimic a condition leading to hypertrophy [32]. In particular, the nucleotides produced by Prosol Spa (Madone BG, Italy) tested in this study includes Ribodiet®, RIBOCARE® MIX 1, RIBOCARE® MIX 2, and RNA-Na.
2.2 Nucleotides mix
All nucleotides used to stimulate the cell were prepared by Prosol Spa (Madone BG, Italy). They are a pool of natural 5'-Nucleotides, obtained by enzymatic hydrolysis of yeast, named Ribodiet®, (5’-AMP.Na2, 5’-CMP.Na2, 5’-UMP.Na2, 5’-GMP.Na2, Nucleosides and other nucleotides); RIBOCARE® MIX 1 is a mix of five 5’ monophosphate nucleotides (5’ AMP free acid, 5’ CMP free acid, 5’ UMP 2 Na, 5’ GMP 2 Na); RIBOCARE® MIX 2 is another mix of four 5’ monophosphate nucleotides (5’ AMP free acid, 5’ CMP free acid, 5’ UMP free acid, 5’ GMP free acid and 5’ IMP free acid); RNA-Na is a ribonucleic acid, sodium salt, obtained from yeast by extraction without organic solvents and neutralized. All the mix were used without solvents and dissolved directly in the medium used to stimulate the cells at a final concentration of 100% (w/v) or 50% (w/v) which corresponded to human dosages of 0.5%/0.6% present in baby milk.
2.3 Cell viability
Cell viability was investigated after at the end of each stimulation time using MTT-based in vitro Toxicology Assay Kit (Sigma-Aldrich, Milan, Italy), as previously described [33]. Briefly, cells were incubated in DMEM without red phenol 0% FBS with 1% MTT dye for 2 h at 37°C in an incubator, and then the formazan crystals were solubilized by Solubilization Solution and the cell viability was determined by measuring the absorbance through a spectrometer (VICTORX4 multilabel plate reader) at 570 nm with correction at 690 nm. The results were obtained comparing them to control cells (100% viable).
2.4 [Mg2+]i Movements
Intracellular Magnesium [Mg2+]i was analyzed using Mg2+-sensitive fluorescent dye Mag-fura-2-AM (Furaptra, Biotium) as previously described [33]. Briefly, the cells were incubated in a Hanks salt solution (Thermo Fisher Scientific, Waltham, USA) without Mg2+ but containing 10 mM glucose and supplemented with 20 mM HEPES/Tris (pH 7.4), 1.3 mM CaCl2, and 5 μM Mag-fura-2-AM at 37°C for 30 min. The fluorescence of cells loaded with Mag-fura-2 was monitored at regular intervals starting from 3min to 300min (15, 30, 60, 120, 150, 180, 210, 240, 270) with excitation at 340 nm and 380 nm. The acquisitions were obtained using a Fluorescence Spectrometer VICTOR X4 at the wavelength of emission of 510 nm with an exposure time of 100 ms. The fluorescence ratios (340/380 nm) were calculated and compared to control; in Rmax and Rmin were analyzed by the addition of 50 mM MgCl2 and 100 mM EDTA, respectively [34].
2.5 [Ca2+] Movements
The analysis of calcium movements was performed following a classical technique [33]. Cells were washed twice with sterile PBS 1× and incubated with 5 μM Fura-2 AM (Sigma-Aldrich, Milan, Italy) for 30 min in the dark in PSS buffer without Ca2+ (1.5 mM KCl, 10 mM HEPES, 10 mM d-Glucose, 2 mM l-Glutamine, pH 7.4), in agitation at 37°C. After the stimulations with different ribonucleotides mix, the fluorescence was measured by the fluorescence spectrometer (VICTOR X4) at the wavelengths of 340 nm for excitation and 510 nm for emission. The results were expressed as a percentage compared to control cells.
2.6 Glucose uptake
After the presence of starved medium (DMEM without red phenol) overnight, cells were stimulated with Ribomix® in presence of 2-deoxyglucose (2-DG), followed by extensive washing three times with KrebseRinger's buffer (20 mM HEPES, 5 mM KH2PO4, 1 mM MgSO4, 1 mM CaCl2, 136 mM NaCl, and 4.7 mM KCl, pH 7.4) following a classical protocol [35] and lysis in the Extraction buffer by several freeze/thaw cycles and then heat at 85°C for 40 min. The cooling cell lysate was put on ice for 5 minutes, neutralized by adding 10 μL of Neutralization Buffer, spun down at 13,000 × g to remove insoluble material and then the amount of glucose in the lysate was measured using Glucose Uptake Colorimetric Assay Kit (Sigma-Aldrich) was performed following the manufacturer’s instruction. After being reacted with mixed reagents A and B, the absorbance of the mixture was measured at 412 nm by spectrophotometer (VICTOR X4). Data were expressed as mean ± SD of pmol/µl derived by standard curve.
2.7 Glycogen measurement
The glycogen synthesis assay was performed as described in the literature [36] and following the manufacturer’s instruction (BioVision, Life Research, Scoresby Victoria, Australia). C2C12 cell lysates were collected and homogenates and boiled for 5 min to inactivate enzymes. Samples were then centrifuged at 13000 rpm for 5 min and the supernatant was pick up to induce the hydrolysis of glycogen to glucose in presence of an OxiRed probe to generate color (lmax = 570 nm). The glycogen concentration in the samples was calculated by C = Ay/Sv where Ay is the amount of glycogen (mg) in the sample as determined from a standard curve and Sv is the sample volume (ml) [36].
2.8 Lactate measurement
As reported in the literature [37] the quantification of lactate level on C2C12 cell was analyzed after lyses on supernatants using a lactate assay kit (BioVision) according to the manufacturer’s protocol. The contents of pyruvate and lactate were normalized to the protein amount.
2.9 ERK Activation Assay
ERK/MAPK and PI3K/Akt activities were measured by the InstantOne™ ELISA (Thermo Fisher, Milan, Italy) cell lysates following the manufacturer’s instructions [38]. Briefly, 50 μL/well of each lysed were tested in InstantOne ELISA microplate strips after 1 h at room temperature on a microplate shaker with the antibody cocktail. In the end, the detection reagent was added for 20 min and then stopped by adding a stop solution. The strips were measured by a spectrometer (VICTOR X4 multilabel plate reader) at 450 nm. The results were expressed as mean absorbance (%) compared to control.
2.10 Phospho-p38/MAPK ELISA test
After stimulations with all nucleotides in the presence or absence of 2.5 mM caffeine, cells were lysed to assess phosphorylation levels of p38 MAPK using a cold Lysis Buffer kit supplemented with protease and phosphatase inhibitors, following the manufacturer’s instruction (p38 MAPK alpha (pT180/pY182) + total p38 MAPK alpha ELISA Kit, Abcam, ab221012) [39]. Absorbance was read at 450 nm on a microplate reader and the results expressed as means (%) vs control.
2.11 Western blot analysis
At the end of each treatment, C2C12 cells were washed twice with ice-PBS 1x and lysed in ice Ripa Buffer (50 mM Hepes, 150 mM NaCl, 0,1% SDS, 1%TRITON 100x, 1% deoxycholate acid, 10% glycerol, 1.5 mM MgCl2, 1 mM EGTA, 1 mM NaF; all purchased from Sigma-Aldrich) supplemented with 2 mM sodium orthovanadate (Sigma-Aldrich), 1 mM phenylmethanesulfonyl fluoride (PMSF; Sigma-Aldrich) and 1:100 mix Protease Inhibitor Cocktail (Sigma-Aldrich).40 μg proteins of each sample were resolved into 8% and 15% SDS-PAGE gels, and polyvinylidene difluoride (PVDF) membranes (GE Healthcare) were incubated overnight at 4°C with specific primary antibody: anti-Cyclin D1 (1:1000, Cell Signalling), anti-Desmin (1:1000, Santa-Cruz), anti-Phospho-AMPK (1:1000, Santa-Cruz), anti-AMPK (1:500, Santa-Cruz), and anti-SMA (1:1000, Santa-Cruz). Protein expression was normalized and verified through β-actin detection (1:5000; Sigma-Aldrich) and expressed as a mean ± SD (% vs control).
2.12 Statistical analysis
Data reported were obtained from at least four independent experiments running on triplicates for each experimental protocol and processed using Prism GraphPad statistical software. Results are expressed as mean ± SD using One-way ANOVA followed by Bonferroni post hoc test for statistical analysis. p values <0.05 were considered statistically significant.
3. Results
3.1 Time-dependent study of cell viability and proliferation on C2C12 Cells
In order to explore the intracellular mechanisms activated by nucleotides, firstly the analysis of mitochondrial health by MTT was carried out. The first set of experiments was performed to demonstrate the effect exerted on cell viability by direct exposure to different nucleotides preparations during the time (ranging from 1h to 7h) and to exclude any cytotoxic effects of the formulations. As shown in Table 1, all nucleotides were able to induce an increase on cell viability during the time; in particular Ribodiet®, Ribocare® MIX 1 and 2, RNA-Na-E showed a time-dependent increase on this parameter with a greater effect starting from 5h to 7h (p<0.05 vs control). Furthermore, the nucleotides RNA-Na-E, which was composed only of RNA, was able to induce a late response on cell viability, supporting the hypothesis of a late effect with respect to other nucleotides. All these data confirmed that none of the forms of nucleotides had cytotoxic effect overtime on muscle cells.
In addition, in order to understand if these nucleotides were also able to induce a physiological differentiation on myotubes of C2C12 cells, additional experiments were carried out stimulating cells for 24h analyzing their proliferation rate. As reported in Figure 1A, the effects of all nucleotides lead to a stable value around 24h of stimulation on cell viability (p<0.05 vs control), since the main effect was observed by Ribocare® MIX 2 stimulation compared to other nucleotides (about 5 round compared to Ribodiet® p<0.05; about 25% compared to Ribocare® MIX 1; about 40% compared to RNA-Na-E). This finding indicated for the first time that nucleotides were able to induce the proliferation and differentiation phases, as confirmed by cyclin D1 and desmin on western blot analysis (Figure 1B and 1C). Indeed, as illustrated in Figure 1B, nucleotide Ribocare® MIX 1 was able to induce a greater effect compared to other nucleotides (p<0.05) confirming that the expression cyclin D1 is abundant in proliferating myoblasts. Similar data were reported by desmin analysis (Figure 1C) in which nucleotide Ribocare® MIX 1 showed the ability to induce greater expression compared to all other nucleotides (p<0.05), confirming a better influence on proliferation of this form.
3.2 Nucleotide-induced contraction mechanisms on C2C12 cells
As is known, muscle contraction during exercise depends on variations in the intracellular concentrations of calcium ions (Ca2+). Given the behavior of calcium in C2C12 cells is similar to that in vivo, changes in cytosolic calcium and magnesium levels in all treatments using Fura-2 and Furaptra, respectively, were studied in these cells. As shown in Figure 2, the administration of all nucleotides was able to modify the calcium movements in a few minutes and this rapid effect was followed by a reduction in intracellular magnesium, supporting the hypothesis of a physiological role exerted by nucleotides. during muscle contraction. This intracellular ion flow cycle was maintained throughout the analyzed period (from 3 'to 300'), indicating a rapid phase of contraction and relaxation, as occurs in the human physiological mechanism.
In order to clarify the balance between the calcium and magnesium movements, a schematic table (Table 2) summarizes the main effects over time of all the nucleotides tested. In particular, stimulation with Ribodiet® was able to maintain a physiological trend compared to other treatments; Ribocare® mix 1 seemed to support exercise better than other nucleotides, inducing rapid contraction of muscle cells; Ribocare® mix 2 appeared to have a similar tendency to Ribocare® mix 1 but its effect was slower than Ribocare® mix 1 (at 30 min), And it confirmed their ability to have a slow effect on muscle cells that have a long relaxing phase. However, it is currently impossible to determine which nucleotide is capable of best supporting exercise.
3.3 Analysis of the intracellular pathways activated by nucleotides on C2C12 cells
To explore whether different nucleotides can support different phases and moments in the intracellular mechanisms involved in an exercise, further experiments were performed by analyzing some important kinases in muscle cells. In particular, the phosphorylation of PI3K/Akt, ERKs/MAPK and 5′ AMP-activated protein kinase (AMPK) which are intracellular signaling proteins typically activated in exercised/contracted skeletal muscle, were investigated. As reported in Figure 3, the activity assay of ERKs/MAPK was analyzed at different times (at 3min and 24h), in order to explore the rapid effects and upregulation caused by a long incubation period. Data reported confirmed its role to help the increases of glucose transport in few mins of stimulation induced by both Ribodiet® and Ribocare® MIX 1 nucleotides compared to Ribocare® MIX 2 and RNA-Na-E (p<0.05) and the greater effects was observed by Ribodiet® (p<0.05 vs all nucleotides), indicating a rapid activation of the intracellular pathway leading to glucose uptake supporting the early phase of exercise. The reported data confirmed the role of ERKs/MAP in facilitating the increase in glucose transport after a few minutes of stimulation induced by both Ribodiet® and Ribocare® MIX 1 nucleotides compared to Ribocare® MIX 2 and RNA-Na-E (p <0.05). The greatest effects were observed with Ribodiet® (p <0.05 vs all other nucleotides), indicating rapid activation of the intracellular pathway leading to rapid glucose uptake to support the initial phase of exercise. In contrast, the 24-hour effects support previous data on the proliferation phase and all nucleotides were able to induce ERK activity. However, the Ribocare® MIX 2 showed better influence than other nucleotides (p <0.05). A second major pathway supporting GLUT4 translocation and glucose uptake, PI3K / Akt (Figure 3B) at 24 hours, confirmed its ability to support a late phase of cell activation, since only formulations Ribocare® MIX 1 and Ribocare® MIX 2 were able to induce its activation (p <0.05). Furthermore, Ribocare® MIX 2 nucleotide induced a more potent effect (approximately 5 times compared to Ribocare® MIX 1, p <0.05). Another key regulator of cellular metabolism which plays a predominant role in catabolic mechanisms: glucose transport and differentiation mechanism of myotubes is AMPK, as reported in Figure 3C at 24h. Nucleotides Ribodiet®, Ribocare® MIX 1 and RNA-Na-E were able to induce greater effects compared to Ribocare® MIX 2 (p<0.05).
Since the exercise in vivo induces a cascade of intracellular signaling (e.g. activation of AMPK), proteomic (e.g. increased GLUT4 expression) and metabolic changes (e.g. enhanced glucose uptake) within skeletal muscle cells additional parameters such as glucose uptake, glycogen consumption and lactate were consequently investigated. As reported in Table 3, the intracellular mechanism reflects what observed by glucose and glycogen analysis; indeed, a rapid glucose uptake, increases glycogen accumulation and increases the rate of extracellular lactate following a physiological mechanism of exercise. In particular, in presence of Ribodiet®, Ribocare® MIX 2 and RNA-Na-E the glucose uptake was significantly increased compared to control (p<0.05). Among these, the best effect seems to be exerted by Ribocare® MIX 2 compared to Ribodiet® (about 11.22%) and RNA-Na-E (about 2.5 times). Glycogen, which is the main intracellular store of glucose, was increased by the stimulation of the cells with all tested nucleotides (p<0.05), indicating that they increased the uptake of glucose to feed the glycogen synthesis process and the main effect was shown by nucleotide Ribocare® MIX 2 (p<0.05 compared to Ribocare® MIX 1 and RNA-Na-E). Finally, lactate, which has an important role in energy metabolism, measured in extracellular environment showed an increase after stimulation with all nucleotides compared to control (p<0.05) and Ribocare® MIX 2 revealed a main effect compared to other nucleotides indicating that this form may be the better choice to support the muscle exercise.
3.4 In vitro exercise simulation by caffeine-induced contraction
It is well established by in vitro studies that caffeine has a direct effect on muscle contraction. It acts on the sarcoplasmic reticulum, increasing calcium permeability and making it readily available for the contraction mechanism [40]. Caffeine treatment has been applied to C2C12 cells with the specific intent to provide an ‘exercise-like treatment’, mimic exercise activation to examine exercise-regulated changes in signal transduction and metabolism. Cells were pre-treated with 2.5 mM caffeine and then treated with all nucleotides in various time points. In order to understand the effects on contraction mechanisms, the levels of calcium and p38/MAPK activity were analyzed. As reported in Figure 4A, all nucleotides tend to dampen calcium oscillations overtime to reach the relaxation phase in the presence of caffeine; indeed, below 2h it was observed all the forms were in resting, at 30 min they tend to contraction again while at 1h the system is rebalanced again (relaxation). This effect was confirmed by the analysis of p38 activity by assay kit (Figure 4B), in which a reduction of contraction was observed starting from 3min up to 2h and then the activity returns to physiological levels starting from 3h up to 5h. In particular, the addition of nucleotide Ribodiet® counteracts caffeine-induced hypercontraction with a better effect compared to Ribocare® MIX 1 (p<0.05). These data demonstrate that nucleotides have different properties during muscular contraction; so that in severe contraction Ribocare® MIX 2 plays a minor role compared to Ribodiet®.
In order to confirm these effects, the analysis of glucose, glycogen and lactate was done after pre-treatment with caffeine and then stimulated with all nucleotides. As shown in Table 4, caffeine alone induced severe contraction accompanied by high glucose uptake and glycogen and a significant reduction on extracellular lactate (p<0.05), indicating a negative condition of the cells which are in hypercontractility state. The addition of single nucleotides was able to reduce this negative condition, leading the extracellular lactate to the control value (p<0.05 vs caffeine alone). Finally, Ribodiet® confirmed to be able to support muscle activity in aerobic physiological conditions.
Since the severe contraction can cause muscle hypertrophy accompanied by cell death when the stress condition is maintaining for a long time, additional experiments were carried out at 24h analyzing cell viability, ERK/MAPK activity and SMA proteins expressions to verify if nucleotide may be also helpful to restore the damage activating cell proliferation. As reported in Figure 5A, a high dose of caffeine caused a decrease in cell viability (p<0.05 vs control) confirming data present in literature and this negative effect was counteracted by all tested nucleotides (p<0.05). However, both Ribodiet® and Ribocare® MIX 2 seem to have greater effects than the other forms, where the former was also able to reduce hyper-contractility and the latter did not counteract the movement of the calcium during contraction, but induced cell proliferation, which could activate survival mechanisms. These findings have been confirmed by ERK activity assay (Figure 5B), in which only Ribodiet® and Ribocare® MIX 2 were able to have the main effects compared to other nucleotides (p<0.05). Furthermore, all nucleotides were able to counteract the negative effect of caffeine alone (p<0.05). Finally, the analysis of regeneration and myogenesis markers such as smooth muscle actin (SMA) showed similar results (Figure 5C). indeed all nucleotides counteracted the negative effect of caffeine and the main effects were observed with Ribodiet® and Ribocare® MIX 2 stimulations (p<0.05) compared to other nucleotides while between Ribodiet® and Ribocare® MIX 2 no significant change was observed, indicating an equal beneficial action on muscle cells in severe contraction conditions. All of these results support the hypothesis that different nucleotides may have different roles during different phases of muscle activity and also reveal different beneficial effects during a strong contraction.
Nucleotides |
1h |
2h |
3h |
4h |
5h |
6h |
7h |
Ribodiet® |
1.33 ± 0.58 |
3.23 ± 3.02 |
1.33 ± 0.57 |
5.09 ± 1.01 |
5.1 ± 1.01* |
10.04 ± 1* |
44.14 ± 2.01* |
Ribocare® Mix 1 |
2.26 ± 1.09 |
2.19 ± 1.05 |
1 ± 0.2 |
1 ± 0.9 |
16.03 ± 1* |
16.5 ± 0.57* |
24.24 ± 2.04* |
Ribocare® Mix 2 |
26.09 ± 2* |
18.22 ± 2.05* |
12.27 ± 2.06* |
13.3* ± 0.58 |
21.20 ± 2.03* |
11.03 ± 2* |
1 ± 0.1 |
RNA-Na-E |
1.33 ± 0.57 |
1.34 ± 0.6 |
1.33 ± 0.5 |
1.33 ± 0.7 |
1.35 ± 0.8 |
55.04 ± 2* |
30.24 ± 1.08* |
Table 1: Cell viability of C2C12 cells stimulated with different nucleotides forms. The data reported are expressed as means ± SD (%) compared to control value (0%). * p<0.05 vs control.

Figure 1: Cell viability and proliferation analysis at 24h of stimulation.
(A) Cell viability measured by MTT test; (B) and (C) Cyclin D1 and desmin expressions measured by western blot, respectively. Data reported are expressed as means ± SD (%) compared to control (0 line) of 4 independent experiments. *p<0.05 vs control; the bars p<0.05 between different nucleotides.

Figure 2: Analysis of calcium and magnesium flux on C2C12 cells.
(A) Calcium and (B) Magnesium flux measured by Fura and Furaptra fluorescence dyes respectively during time (starting from 3 min to 5h). Data reported are expressed as means ± SD (%) of four experiments vs control (0 line).
Ribodiet® |
3’ |
15’ |
30’ |
60’ |
120’ |
250’ |
180’ |
210’ |
240’ |
270’ |
300’ |
C |
R |
C |
R |
C |
C |
C |
R |
R |
C |
R |
|
Ribocare® Mix 1 |
C |
C |
C |
R |
R |
C |
C |
C |
C |
C |
R |
Ribocare® Mix 2 |
S |
S |
C |
R |
R |
C |
C |
R |
R |
C |
C |
RNA-Na-E |
S |
C |
C |
R |
R |
R |
C |
C |
S |
R |
R |
C=contraction; R=relaxation; S=resting phase.
Table 2: Analysis of the balance between calcium and magnesium flux under nucleotides stimulations on C2C12 cells. The empirical analysis summarized the effects observed on Figure 1 and the conclusion of each time point was obtained by the ratio from calcium and magnesium (who is big the other correspond to the main effect observed).
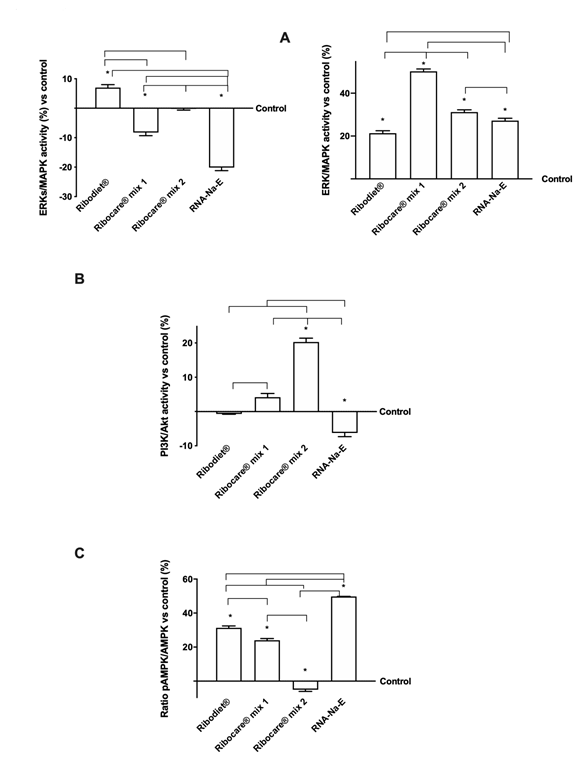
Figure 3: ELISA activity and western blot analysis. (A) and (B) ELISA test of ERKs/MAPK activity measured at 3min (on the left) and 24h (on the right) and PI3K/Akt at 24h measured after stimulation with all nucleotides tested. Data are expressed as means ± SD of four experiments compared to control (0% line); (C) Western blot and densitometric analysis of the AMPK expression. The images shown are an example of each protein from four independent experiments. Data are expressed as means ± SD (%) normalized on total protein and verified on β-actin detection.
CTR |
Glycogen microg/microl |
Extracell. Lactate nmol/microl |
Glucose pmol/microl |
0.639 ± 0.01 |
142 ± 0.03 |
36.27 ± 1.62 |
|
Ribodiet® |
0.745 ± 0.01* |
142.1 ± 0.02* |
42.42 ± 2.66* |
Ribocare® Mix 1 |
0.692 ± 0.02* |
141.2 ± 0.03* |
38.09 ± 1.65 |
Ribocare® Mix 2 |
0.752 ± 0.03* |
143.7 ± 0.04* |
47.18 ± 3.02* |
RNA-Na-E |
0.693 ± 0.01* |
141 ± 0.02* |
18.30 ± 2.07* |
Table 3: Analysis of Glucose uptake, glycogen and lactate by ELISA test. Data reported are expressed as means ± SD of four independent experiments carried out in triplicates. CTR= control sample. *p<0.05 vs control.
CTR |
Glycogen microg/microl |
Extracell. Lactate nmol/microl |
Glucose pmol/microl |
0.66 ± 0.024 |
143.6 ± 1.51 |
34.27 ± 2.05 |
|
Caffeine + Ribodiet® |
0.93 ± 0.01* |
144.3 ± 0.06 |
30.97 ± 0.12* |
Caffeine + Ribocare® Mix 1 |
0.64 ± 0.5 |
145.3 ± 0.62 |
30.50 ± 0.3 |
Caffeine + Ribocare® Mix 2 |
0.129 ± 0.11* |
146.9 ± 1.78 |
20.54 ± 1.33* |
Caffeine + RNA-Na-E |
0.74 ± 0.5* |
143.1 ± 0.1 |
25.53 ± 0.16* |
Caffeine |
2.08 ± 0.03* |
120.7 ± 2.53* |
66.73 ± 2.61* |
Table 4: Analysis of Glucose uptake, glycogen and lactate by ELISA test. Data reported are expressed as means ± SD of four independent experiments carried out in triplicates. CTR= control sample. * p<0.05 vs control.
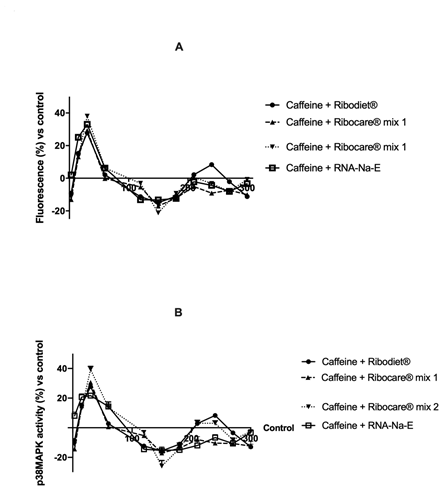
Figure 4: Calcium movement and p38/MAPK activity analyzed in presence of 2.5 mM caffeine.
(A) Calcium fluorescence and (B) p38 activity. Data are expressed as means ± SD (%) compared to control value (0 line) of four independent experiments.
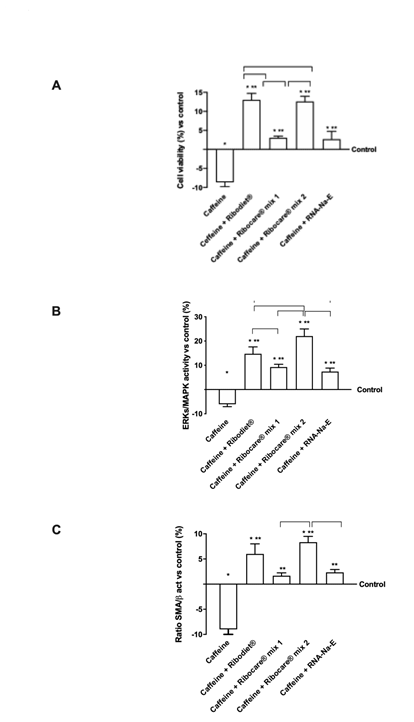
Figure 5: Cell viability and protein activated by nucleotides in presence of caffeine. (A) Cell viability measured by MTT test; (B) ERK/MAPK activity measured by ELISA test; (C) Western blot and densitometric analysis. Data reported are expressed as means ± SD of four independent experiments expressed as % vs control (0 line). Western blot reported is an example and Data are expressed as means ± SD (%) normalized on total protein and verified on β-actin detection. * p<0.05 vs control; ** p<0.05 vs caffeine; the bars p<0.05 between different nucleotides.
4. Discussion
In this study, conducted on muscle cells, the intracellular mechanisms evoked by the main nucleotides used as food supplements were verified for the first time. The data obtained made it possible to identify for the first time two important roles they played; in physiological conditions, the tested nucleotides can support and improve the energy balance of the cell and in conditions of intense physical exercise they support a prevention mechanism capable of counteracting the damage caused by severe hypercontraction conditions. In particular, different effects of Ribodiet®, Ribocare® mix 1 and 2 were observed with respect to RNA-Na. In fact, in physiological operating conditions starting from the analyzed parameters Ribocare® MIX 2 was found to have a greater effect than other nucleotides. However, Ribodiet® seems to play a major role during the first phase of the exercise compared to Ribocare® MIX 2. On the other hand, Ribocare® MIX 2 and Ribodiet® play a similar role under intense exercise conditions by activating different damage restoration mechanisms (both SMA protein and cell differentiation).
A strength of this study was the use of an in vitro model of physical exercise [41], through the administration of caffeine, which allowed to investigating the cellular effects of individual aspects of the physiology of exercises, such as the contraction of the skeletal muscle or the activation of signaling pathways that respond to exercise, directly on skeletal muscle cells, as well as exercise-mediated cross-talk between different cell types [42]. C2C12 cells have been extensively used to study the effects of various agents not only in myoblasts, which are an undifferentiated state of myogenic differentiation, but also in myotubes, a differentiated state on insulin-dependent or independent mechanisms of glucose uptake and expression of GLUT-4 [43].
Skeletal muscle is an absolutely important element for relational life as it supports physical activity that allows us to interact with the environment around us [44] and it is the largest tissue in the human body, since it represents up to 40-50% of body weight [43]. Although there has been a lot of in-depth research on exercise in recent years, the many human health benefits of controlling muscle tissue still remain to be fully understood. Such benefits could be improved glucose disposal, changes in muscle fiber types, induction of angiogenesis, and regulation of the immune system in both the prevention and treatment of various chronic diseases [45, 46]. However, over the course of a lifetime, the trophism of muscle tissue can undergo profound changes, such as skeletal muscle hypertrophies that occur following intense resistance training or atrophies that occur rapidly, for example during prolonged bed rest [47-49]. Also, another important factor is that skeletal muscle mass and strength decrease with age, which can lead to decreased levels of physical activity and increased risk of falling with consequent negative effects that affect the quality of life [44]. Moreover, the exact intracellular mechanisms responsible for the benefits of exercise remain to be determined. Indeed, skeletal muscle contraction per se likely contributes to some or many of the exercise benefits, be that through direct effects within the contracting skeletal muscle cells, such as improved glucose uptake and insulin sensitivity [50, 51] or mediated by factors released from the skeletal muscle cells, such as myokines and/or metabolites [52, 53]. Determining key mechanisms and signal transduction specific to each exercise-triggered benefit could optimize its beneficial role in maintaining health, minimizing impairment of exercise adaptation, and could facilitate the identification of novel drug targets responsive to exercise. In this context, many important data on the role of nutrition in the regulation of skeletal muscle mass are reported in the literature. Recent studies have reported the importance of nutrition-induced regulation of mRNA levels for a range of metabolic genes [44]. For example, Anthony et al. reported that the oral administration of leucine stimulated protein synthesis in skeletal muscles [54], or Yoshihara et al. that the administration of 6% beef extract for 4weeks increased leg muscle weight and modified the muscle fiber type [55]. Furthermore, the consumption of dietary supplements with “ergogenic” claims occurs in numerous target categories, such as athletes [56], the elderly [57], patients with chronic diseases [58], and sedentary adults [59]. The influence of plants-based food supplements in improving exercise performance has been a focus of many studies, in order to explain the main mechanism of every single ingredient. Indeed, Antioxidant phytochemical supplements are used by physically active individuals as ergogenic functional foods for improving physiological or metabolic responses that may increase performance during exercise [60]. High-intensity exercise training leads to excessive levels of reactive oxygen species, pro-inflammatory cytokines, and excess concentration of cortisol which may hamper sports performance, induce fatigue, and delay recovery [61]. Therefore, developing antioxidant phytochemicals to prevent exercise-induced oxidative stress along with improving adaptation responses to endurance training is an attractive strategy for physically active individuals [62]. Thus, it is necessary to study in-depth the ever-growing area of nutrients/nutraceuticals regarding various aspects of skeletal muscle health and associated cellular mechanisms to formulate evidence-based recommendations [63]. In this context, our study may suggest a possible new intervention strategy through dietary supplementation capable of acting on physical exercise, using a natural compound, nucleotides. The use of nucleotides is characterized by a greater compliance for muscle cells, since they are very important for mitochondrial function by promoting many functions of muscle cells. C2C12 cells, the mouse skeletal muscle cell line, were used for this. Although in vivo experiments with human volunteers represent a more integrated model for examining responses to exercise, a preemptive examination of the mechanisms from a molecular point of view is needed to optimize experimental models to resemble real elements of the physiology of exercise, but with a more reductionist approach [42].
5. Conclusion
In conclusion, this study lays the foundations for the development of a new strategy, based on nucleotides dietary supplements, which can provide beneficial effects on muscle function, whether during physical exercise or when recovering from an exercise or to counteract the physiological process of muscle mass loss in the elderly.
Statement of Competing Interests
The authors have no competing interests.
Acknowledgements
The authors thanks Prosol SPA which have donated nucleotides. This study was (partially) funded by the AGING Project-Department of Excellence-Department of Translational Medicine, Università del Piemonte Orientale.
References
- Moriura T, Matsuda H, Kubo M. Pharmacological study on Agkistrodon blomhoffii blomhoffii BOIE. V. anti-fatigue effect of the 50% ethanol extract in acute weight-loaded forced swimming-treated rats. Biological and Pharmaceutical Bulletin 19 (1996): 62-66.
- Kim KM, Yu KW, Kang DH, et al. Anti-stress and anti-fatigue effects of fermented rice bran. Bioscience Biotechnology Biochemistry 65 (2001): 2294-2296.
- Tan W, Yu KQ, Liu YY, et al. Anti-fatigue activity of polysaccharides extract from Radix Rehmanniae Preparata. International Journal of Biological Macromolecules 50 (2012): 59-62.
- Azizbeigi K, Stannard SR, Atashak S, et al. Antioxidant enzymes and oxidative stress adaptation to exercise training: comparison of endurance, resistance, and con-current training in untrained males. Journal of Exercise Science and Fitness 12 (2014): 1-6.
- Echtay KS, Roussel D, St-Pierre J, et al. Superoxide activates mitochondrial uncoupling proteins. Nature 415 (2002): 96-99.
- Wang X, Xing R, Chen Z, et al. Effect and mechanism of mackerel (Pneumatophorus japonicus) peptides for anti-fatigue. Food & Function 5 (2014): 2113-2119.
- Lee JS, Kim HG, Han JM, et al. Anti-fatigue effect of Myelophil in a chronic forced exercise mouse model. European Journal of Pharmacology 764 (2015): 100-108.
- Chi A, Li H, Kang C, et al. Anti-fatigue activity of a novel polysaccharide conjugates from Ziyang green tea. International Journal of Biological Macromolecules 80 (2015): 566-572.
- Ostojic SM, Idrizovic K, Stojanovic MD. Sublingual nucleotides prolong run time to exhaustion in young physically active men. Nutrients 5 (2013): 4776-4785.
- Martinez-Puig D, Manzanilla EG, Morales J, et al. Dietary nucleotide supplementation reduces occurrence of diarrhoea in early weaned pigs. Livestock Science 108 (2007): 276-279.
- Cai X, Bao L, Wang N, et al. Dietary nucleotides supplementation and liver injury in alcohol-treated rats: a metabolomics investigation. Molecules 21 (2016): 435.
- Cai X, Bao L, Wang N, et al. Dietary nucleotides protect against alcoholic liver injury by attenuating inflammation and regulating gut microbiota in rats. Food Function 7 (2016): 2898-2908.
- Xu M, Zhao M, Yang R, et al. Effect of dietary nucleotides on immune function in Balb/C mice. Int Immunopharmacology 17 (2013): 50-56.
- Xu M, Liang R, Guo Q, et al. Dietary nucleotides extend the life span in Sprague-Dawley rats. The Journal of Nutrition, Health and Aging 17 (2013): 223-229.
- Hess JR, Greenberg NA. The Role of Nucleotides in the Immune and Gastrointestinal Systems: Potential Clinical Applications. Nutrition in Clinical Practice 27 (2012): 281-294.
- Sánchez-Pozo A, Gil A. Nucleotides as semiessential nutritional components. Br J Nutr 87 Suppl 1 (2002): S135-137.
- Verkerk R. Nucleotides: Speculation on lifestyle-Induced Essentiality. NHD - The magazine for Dietitians, Nutritionists and Healthcare Professionals 67 (2011): 29-32.
- Koletzko B, Baker S, Cleghorn G, et al. Global Standard for the Composition of Infant formula: Recommendations of an ESPGHAN Coordinated International Expert Group. Journal of Pediatric Gastroenterology and Nutrition 41 (2005): 584-599.
- Dancey C, Attree E, Brown K. Nucleotide supplementation: a randomised double-blind placebo controlled trial of IntestAidIB in people with Irritable Bowel Syndrome. Nutrition Journal 5 (2006): 16.
- Jyonouchi H (1994) Nucleotide actions on the humoral immune response. Journal of Nutrition 124 (1994): 138-143.
- Belo A, Marchbank T, Fitzgerald AJ, et al. Gastroprotective effects of oral nucleotide administration. Gut 55 (2006): 165-171.
- Uauy R. Dietary nucleotides and requirements in early life. In Ed.: Lebenthal E. Textbook of gastroenterology and nutrition in infancy, Raven Press, Ltd, New York, USA (1990): 265-280.
- Hawkes JS, Gibson RA, Roberton D, et al. Effect of dietary nucleotide supplementation on growth and immune function in term infants: a randomized controlled trial. European Journal of Clinical Nutrition 60 (2006): 254-264.
- Davidson I, Fyfe I. A Randomised Double Placebo Controlled Trial of Nucleotide-Containing Supplement Nucell on Symptoms of Participants with the Common Cold-A Pilot Study. EC Nutrition 1 (2016): 804-811.
- Matsumoto Y, Adjei AA, Yamauchi K, et al. Nucleoside-nucleotide mixture increases peripheral neutrophils in cyclophosphamide-induced neutropenic mice. Nutrition 11 (1995): 296-299.
- Xu M, Liang R, Li Y, et al. Anti-fatigue effects of dietary nucleotides in mice, Food and Nutrition Research 61 (2017): 1.
- Salobir J, Rezar V, Pajk, T, et al. Effect of nucleotide supplementation on lymphocyte DNA damage induced by dietary oxidative stress in pigs. Animal Science 81 (2005): 135-140.
- Mc Naughton L, Bentley DJ, Koeppel P. The effects of a nucleotide supplement on salivary IgA and cortisol after moderate endurance exercise. The Journal of Sports Medicine and Physical Fitness 46 (2006): 84-89.
- Schwellnus MP, Derman EW, Noakes TD. Etiology of skeletal muscle 'cramps' during exercise: a novel hypothesis. Journal of Sports Sciences 15 (1997): 277-285.
- Mehta SS, Fallon MB. Muscle Cramps in Liver Disease. Clinical Gastroenterology and Hepatology 11 (2013): 1385-1391.
- Patent_ A61K31/7004 - Monosaccharides having only carbon, hydrogen and oxygen atoms.
- Park S, Scheffler TL, Gunawan AM, et al. Chronic elevated calcium blocks AMPK-induced GLUT-4 expression in skeletal muscle. American Journal of Physiology-Cell Physiology 296 (2009): 106-115.
- Uberti F, Morsanuto V, Ruga S, et al. Study of Magnesium Formulations on Intestinal Cells to Influence Myometrium Cell Relaxation. Nutrients 12 (2020): 573.
- Gouadon E, Lecerf F, German-Fattal M. Differential effects of cyclosporin A and tacrolimus on magnesium influx in Caco-2 cells. Journal of Pharmacy and Pharmaceutical Sciences 15 (2012): 389-439.
- Tsuka S, Aonuma F, Higashi S, et al. Promotion of insulin-induced glucose uptake in C2C12 myotubes by osteocalcin. Biochemical and Biophysical Research Communications 459 (2015): 437-442.
- Myers SA, Nield A, Chew GS, et al. The zinc transporter, Slc39a7 (Zip7) is implicated in glycaemic control in skeletal muscle cells. PLoS One 8 (2013): e79316.
- Hong J, Kim BW, Choo HJ, et al. Mitochondrial complex I deficiency enhances skeletal myogenesis but impairs insulin signaling through SIRT1 inactivation. Journal of Biological Chemistry 289 (2014): 20012-20025.
- Uberti F, Morsanuto V, Aprile S, et al. Biological effects of combined resveratrol and vitamin D3 on ovarian tissue. Journal of Ovarian Research 10 (2017): 61.
- Gartz M, Darlington A, Zeeshan Afzal M, et al. Exosomes exert cardioprotection in dystrophin-deficient cardiomyocytes via ERK1/2-p38/MAPK signaling. Scientific Reports 8 (2018): 16519.
- Weber A. The mechanism of the action of caffeine on sarcoplasmic reticulum. Journal of General Physiology 52 (1968): 760-772.
- Chen W, Nyasha MR, Koide M, et al. In vitro exercise model using contractile human and mouse hybrid myotubes. Scientific Reports 9 (2019): 11914.
- Carter S, Solomon TPJ. In vitro experimental models for examining the skeletal muscle cell biology of exercise: the possibilities, challenges and future developments. Pflügers Archiv European Journal of Physiology 471 (2019): 413-429.
- Ma J, Meng X, Kang SY, et al. Regulatory effects of the fruit extract of Lycium chinense and its active compound, betaine, on muscle differentiation and mitochondrial biogenesis in C2C12 cells. Biomedicine and Pharmacotherapy 118 (2019): 109297.
- Sawano S, Baba K, Sonoda Y, et al. Beef extract supplementation promotes myoblast proliferation and myotube growth in C2C12 cells. European Journal of Nutrition (2020).
- Jessen N, Goodyear LJ. Contraction signaling to glucose transport in skeletal muscle. Journal of Applied Physiology 99 (2005): 330-337.
- Nedachi T, Fujita H, Kanzaki M. Contractile C2C12 myotube model for studying exercise-inducible responses in skeletal muscle. American Journal of Physiology-Endocrinology and Metabolism 295 (2008): 1191-1204.
- Gollnick P, Piehl K, Saubert C, et al. Diet, exercise, and glycogen changes in human muscle fibers. Journal of Applied Physiology 33 (1972): 421-425.
- Higbie EJ, Cureton KJ, Warren GL, et al. Effects of concentric and eccentric training on muscle strength, cross-sectional area, and neural activation. Journal of Applied Physiology 81 (1996): 2173-2181.
- Berg H, Larsson L, Tesch P. Lower limb skeletal muscle function after 6 week of bed rest. Journal of Applied Physiology 82 (1997): 182-188.
- Maarbjerg SJ, Sylow L, Richter EA. Current understanding of increased insulin sensitivity after exercise - emerging candidates. Acta Physiology (Oxford) 202 (2011): 323-335.
- Richter EA, Hargreaves M. Exercise, GLUT4, and skeletal muscle glucose uptake. Physiological Reviews 93 (2013): 993-1017.
- Pedersen BK, Febbraio MA. Muscle as an endocrine organ: focus on muscle-derived interleukin-6. Physiological Reviews 88 (2008): 1379-1406.
- Pedersen BK, Febbraio MA. Muscles, exercise and obesity: skeletal muscle as a secretory organ. Nature Reviews Endocrinology 8 (2012): 457-465.
- Anthony JC, Anthony TG, Kimball SR, et al. Orally administered leucine stimulates protein synthesis in skeletal muscle of postabsorptive rats in association with increased eIF4F formation. Journal of Nutrition 130 (2000): 139-145.
- Yoshihara H, Wakamatsu J, Kawabata F, et al. Beef extract supplementation increases leg muscle mass and modifies skeletal muscle fiber types in rats. Journal of Nutritional Science and Vitaminology 52 (2006): 183-193.
- Petróczi A, Naughton DP, Pearce G, et al. Nutritional supplement use by elite young UK athletes: fallacies of advice regarding efficacy. Journal of the International Society of Sports Nutrition 5 (2008): 22.
- Bosaeus I, Rothenberg E. Nutrition and physical activity for the prevention and treatment of age-related sarcopenia. Proceedings of the Nutrition Society 75 (2016): 174-180.
- Fetterman JW, Zdanowicz MM. Therapeutic potential of n-3 polyunsaturated fatty acids in disease. American Journal of Health-System Pharmacy 66 (2009): 1169-1179.
- Robinson MM, Turner SM, Hellerstein MK, et al. Long-term synthesis rates of skeletal muscle DNA and protein are higher during aerobic training in older humans than in sedentary young subjects but are not altered by protein supplementation. FASEB Journal 25 (2011): 3240-3249.
- Williams MH. Dietary Supplements and Sports Performance: Introduction and Vitamins”. Journal of the International Society of Sports Nutrition 1.2 (2004): 1-6.
- He F, Li J, Liu Z, et al. Redox Mechanism of Reactive Oxygen Species in Exercise. Frontiers in Physiology 7 (2016): 486.
- Juturu V, Nair SVG, Ghanam K, et al. Lutein and Zeaxanthin Isomers Induces Mitochondrial Biogenesis and Improves Endurance Capacity in Muscle Cells. EC Ophthalmology 9.9 (2018): 658-668.
- Deane CS, Wilkinson DJ, Phillips BE, et al. "Nutraceuticals" in relation to human skeletal muscle and exercise. American Journal of Physiology: Endocrinology and Metabolism 312 (2017): E282-E299.