Chloride Ion Transport Blockade Enhances the Cytocidal Effects of Sterile Water on Bladder Cancer Cells
Article Information
Yuki Matsuoka1, Rikiya Taoka1*, Yoichiro Tohi1, Xia Zhang1, and Mikio Sugimoto1
Affiliation:
1Department of Urology, Faculty of Medicine, Kagawa University, 1750-1 Ikenobe, Miki-cho, Kita-gun, Kagawa 761-0793, Japan
*Corresponding author:
Rikiya Taoka, Department of Urology, Faculty of Medicine, Kagawa University 1750-1 Ikenobe, Miki-cho, Kita-gun, Kagawa 761-0793, Japan
Received: November 26, 2023; Accepted: December 08, 2023; Published: December 22, 2023
Citation: Yuki Matsuoka, Rikiya Taoka, Yoichiro Tohi, Xia Zhang, and Mikio Sugimoto. Chloride Ion Transport Blockade Enhances the Cytocidal Effects of Sterile Water on Bladder Cancer Cells. Journal of Cancer Science and Clinical Therapeutics 7 (2023): 271-276.
View / Download Pdf Share at FacebookAbstract
We investigated whether 5-nitro-2-(3-phenylpropylamino)-benzoic acid (NPPB), a Cl¬ channel blocker, enhanced the cytocidal effects of hypotonic shock on bladder cancer cell lines. RT112 cells demonstrated limited hypotonic swelling with reduced cell rupture. After NPPB treatment, RT112 cells ruptured faster than control cells. After 10 min of sterile water exposure, the proportions of viable RT112 cells treated with DMSO, and 50, 100, 200, and 300 µM NPPB were 13.6±3.4%, and 6.3±1.2%, 2.0±1.1%, 0.7±0.7%, and 0%, respectively. These results suggest that intravesical therapy using sterile water with a Cl- channel blocker represents a potential new adjuvant therapy for bladder cancer.
Keywords
Bladder cancer, Chloride, Cytocidal effect, Hypotonic shock.
Bladder cancer articles; Chloride articles; Cytocidal effect articles; Hypotonic shock articles.
Bladder cancer articles Bladder cancer Research articles Bladder cancer review articles Bladder cancer PubMed articles Bladder cancer PubMed Central articles Bladder cancer 2023 articles Bladder cancer 2024 articles Bladder cancer Scopus articles Bladder cancer impact factor journals Bladder cancer Scopus journals Bladder cancer PubMed journals Bladder cancer medical journals Bladder cancer free journals Bladder cancer best journals Bladder cancer top journals Bladder cancer free medical journals Bladder cancer famous journals Bladder cancer Google Scholar indexed journals Chloride articles Chloride Research articles Chloride review articles Chloride PubMed articles Chloride PubMed Central articles Chloride 2023 articles Chloride 2024 articles Chloride Scopus articles Chloride impact factor journals Chloride Scopus journals Chloride PubMed journals Chloride medical journals Chloride free journals Chloride best journals Chloride top journals Chloride free medical journals Chloride famous journals Chloride Google Scholar indexed journals Cytocidal effect articles Cytocidal effect Research articles Cytocidal effect review articles Cytocidal effect PubMed articles Cytocidal effect PubMed Central articles Cytocidal effect 2023 articles Cytocidal effect 2024 articles Cytocidal effect Scopus articles Cytocidal effect impact factor journals Cytocidal effect Scopus journals Cytocidal effect PubMed journals Cytocidal effect medical journals Cytocidal effect free journals Cytocidal effect best journals Cytocidal effect top journals Cytocidal effect free medical journals Cytocidal effect famous journals Cytocidal effect Google Scholar indexed journals Hypotonic shock articles Hypotonic shock Research articles Hypotonic shock review articles Hypotonic shock PubMed articles Hypotonic shock PubMed Central articles Hypotonic shock 2023 articles Hypotonic shock 2024 articles Hypotonic shock Scopus articles Hypotonic shock impact factor journals Hypotonic shock Scopus journals Hypotonic shock PubMed journals Hypotonic shock medical journals Hypotonic shock free journals Hypotonic shock best journals Hypotonic shock top journals Hypotonic shock free medical journals Hypotonic shock famous journals Hypotonic shock Google Scholar indexed journals 3-phenylpropylamino articles 3-phenylpropylamino Research articles 3-phenylpropylamino review articles 3-phenylpropylamino PubMed articles 3-phenylpropylamino PubMed Central articles 3-phenylpropylamino 2023 articles 3-phenylpropylamino 2024 articles 3-phenylpropylamino Scopus articles 3-phenylpropylamino impact factor journals 3-phenylpropylamino Scopus journals 3-phenylpropylamino PubMed journals 3-phenylpropylamino medical journals 3-phenylpropylamino free journals 3-phenylpropylamino best journals 3-phenylpropylamino top journals 3-phenylpropylamino free medical journals 3-phenylpropylamino famous journals 3-phenylpropylamino Google Scholar indexed journals Cl¬ channel blocker articles Cl¬ channel blocker Research articles Cl¬ channel blocker review articles Cl¬ channel blocker PubMed articles Cl¬ channel blocker PubMed Central articles Cl¬ channel blocker 2023 articles Cl¬ channel blocker 2024 articles Cl¬ channel blocker Scopus articles Cl¬ channel blocker impact factor journals Cl¬ channel blocker Scopus journals Cl¬ channel blocker PubMed journals Cl¬ channel blocker medical journals Cl¬ channel blocker free journals Cl¬ channel blocker best journals Cl¬ channel blocker top journals Cl¬ channel blocker free medical journals Cl¬ channel blocker famous journals Cl¬ channel blocker Google Scholar indexed journals hypotonic shock articles hypotonic shock Research articles hypotonic shock review articles hypotonic shock PubMed articles hypotonic shock PubMed Central articles hypotonic shock 2023 articles hypotonic shock 2024 articles hypotonic shock Scopus articles hypotonic shock impact factor journals hypotonic shock Scopus journals hypotonic shock PubMed journals hypotonic shock medical journals hypotonic shock free journals hypotonic shock best journals hypotonic shock top journals hypotonic shock free medical journals hypotonic shock famous journals hypotonic shock Google Scholar indexed journals cytocidal effects articles cytocidal effects Research articles cytocidal effects review articles cytocidal effects PubMed articles cytocidal effects PubMed Central articles cytocidal effects 2023 articles cytocidal effects 2024 articles cytocidal effects Scopus articles cytocidal effects impact factor journals cytocidal effects Scopus journals cytocidal effects PubMed journals cytocidal effects medical journals cytocidal effects free journals cytocidal effects best journals cytocidal effects top journals cytocidal effects free medical journals cytocidal effects famous journals cytocidal effects Google Scholar indexed journals muscularis propria articles muscularis propria Research articles muscularis propria review articles muscularis propria PubMed articles muscularis propria PubMed Central articles muscularis propria 2023 articles muscularis propria 2024 articles muscularis propria Scopus articles muscularis propria impact factor journals muscularis propria Scopus journals muscularis propria PubMed journals muscularis propria medical journals muscularis propria free journals muscularis propria best journals muscularis propria top journals muscularis propria free medical journals muscularis propria famous journals muscularis propria Google Scholar indexed journals Intravesical Bacillus Calmette–Guérin articles Intravesical Bacillus Calmette–Guérin Research articles Intravesical Bacillus Calmette–Guérin review articles Intravesical Bacillus Calmette–Guérin PubMed articles Intravesical Bacillus Calmette–Guérin PubMed Central articles Intravesical Bacillus Calmette–Guérin 2023 articles Intravesical Bacillus Calmette–Guérin 2024 articles Intravesical Bacillus Calmette–Guérin Scopus articles Intravesical Bacillus Calmette–Guérin impact factor journals Intravesical Bacillus Calmette–Guérin Scopus journals Intravesical Bacillus Calmette–Guérin PubMed journals Intravesical Bacillus Calmette–Guérin medical journals Intravesical Bacillus Calmette–Guérin free journals Intravesical Bacillus Calmette–Guérin best journals Intravesical Bacillus Calmette–Guérin top journals Intravesical Bacillus Calmette–Guérin free medical journals Intravesical Bacillus Calmette–Guérin famous journals Intravesical Bacillus Calmette–Guérin Google Scholar indexed journals
Article Details
Introduction
Bladder cancer is the ninth-most common cancer worldwide, with 390,000 new cases diagnosed and 150,000 deaths annually [1]. Approximately 75% of diagnosed patients exhibit non-muscle invasive bladder cancer (NMIBC). During the progression of NMIBC, 50% of patients exhibit recurrence, among which 9% of cases show invasion of the muscularis propria [2]. Radical cystectomy represents the standard therapy for muscle invasive bladder cancer. Intravesical Bacillus Calmette–Guérin (BCG) treatment after transurethral resection of bladder tumor (TURBT) reduces the risk of intravesical recurrence in patients with high-risk NMIBC [3-5]. Intravesical BCG treatment is more effective than intravesical chemotherapy [6, 7]. Therefore, the European Association of Urology guidelines recommend intravesical BCG treatment after TURBT in patients with high-risk NMIBC [8]. However, intravesical BCG treatment is associated with more side effects than those associated with intravesical chemotherapy. Notably, 19% of patients who underwent intravesical BCG treatment were required to terminate therapy owing to the associated side effects, as reported in the European Organization for the Research and Treatment of Cancer Genito- Urinary Group trial 30911 [7]. Moreover, the current shortage of BCG is a global concern that has resulted in increasing demand and supply constraints. Therefore, novel intravesical therapies with high efficacy and low toxicity are sought after. We previously demonstrated the cytocidal effect of sterile water on bladder cancer cells [9, 10]. Although sterile water may represent a new agent for intravesical therapy, bladder cancer cell lines differ in their sensitivity to hypotonic shock induced by sterile water. Therefore, future studies should focus on detailed analyses of the cytocidal effects of hypotonic shock for successful clinical application. In this study, we treated bladder cancer cells with 5-nitro-2-(3-phenylpropylamino)-benzoic acid (NPPB), a chloride (Cl-) channel blocker, because Cl- outflow via Cl- channels is necessary for the regulation of cell volume during hypotonic shock. The findings demonstrate the efficacy of intravesical therapy using sterile water after TURBT, and the enhancing effects of NPPB.
Materials and Methods
Cell culture
The human bladder cancer cell lines, RT112 (low-grade), T24 (high-grade), and J82 (high-grade), were obtained from the American Type Culture Collection (Manassas, VA, USA). These adherent cell lines were maintained at 37 °C and 5% CO2 in a humidified atmosphere in RPMI-1640 (Wako, Osaka, Japan) supplemented with 10% fetal bovine serum (Sigma–Aldrich, St. Louis, MO, USA), HEPES buffer (Sigma–Aldrich), and penicillin–streptomycin (Thermo Fisher Scientific, Waltham, MA, USA).
Morphological changes in bladder cancer cells after
exposure to sterile water
After culturing bladder cancer cells in T75 flasks, the culture medium was completely removed and sterile water was added. For the NPPB experiments, the cells were pre- incubated with culture medium containing 50, 100, 200, or 300 µM NPPB for 30 min at 37 °C and 5% CO2 in a humidified atmosphere. The flasks were mounted on the stage of a KEYENCE BZ-9000 All-in-One Fluorescence Microscope (Osaka, Japan), and changes in the cells were recorded at 0, 1, 3, 5, and 10 min.
Cell viability
Cells were plated in 96-well plates at a density of 5 × 103 cells/well. After 24 h of incubation, the cells were exposed to sterile water for 1, 3, 5, or 10 min, after which the water was replaced with culture medium. In the control group, the medium was replaced at the same time. For the NPPB experiments, cells were incubated with culture medium containing 50, 100, 200, or 300 µM NPPB for 30 min at 37 °C and 5% CO2 in a humidified atmosphere prior to treatment with sterile water as described above. After 24 h, cell viability was assessed by pulsing the cells for 2 h with dimethyl thiazolyl diphenyl tetrazolium (MTT; 5 mg/mL in phosphate-buffered saline), followed by solubilization of the formazan crystals in 100 μL of lysis buffer containing 20% sodium dodecyl sulfate and 50% dimethylformamide; the absorbance at 570 nm was measured using a spectrophotometer. All measurements were performed in quadruplicate, and data are presented as means ± standard error of the mean.
Analysis of cell membranes
Bladder cancer cells were detached from the culture flasks using trypsin-EDTA and centrifuged. The pellets were resuspended in 5 mL of medium and the cell suspension was aliquoted into five tubes. After centrifugation, the pelleted cells were resuspended in sterile water and incubated for 1, 3, 5, or 10 min. For the NPPB experiments, cells were incubated with culture medium containing 50, 100, 200, or 300 µM NPPB for 30 min at 37 °C and 5% CO2 in a humidified atmosphere prior to treatment with sterile water as described above. The suspensions were added to LUNA-II™ (Logos Biosystems, Gyeonggi-do, South Korea), and the percentage of intact cell membranes was determined using the trypan blue-exclusion method. All analyses were performed in triplicate, and the data are presented as means ± standard error of the mean.
Statistical analysis
All analyses were performed in triplicates and results are shown as mean ± SEM. Student’s t-test was used to compare the statistical significance of differences. Statistical analyses were performed using SPSS V25.0 (Armonk, NY: IBM Corp.).
Results
Morphological changes were observed in the bladder cancer cell lines after exposure to sterile water (Figure 1). T24 and J82 cells started swelling as soon as they had been exposed to sterile water; they continued swelling until they ruptured. The rupturing occurred within 3–5 min of their exposure to sterile water. RT112 cells demonstrated limited hypotonic swelling with significantly less cell ruptures after 10 min. However, RT112 cells treated with high concentrations of NPPB ruptured faster than cells treated with dimethyl sulfoxide (DMSO; Figure 2). Next, we performed the MTT assay to analyze bladder cancer cell viability after exposure to sterile water (Figure 3). In each of the bladder cancer cell lines, the decrease in cell viability was dependent on the duration of exposure to sterile water. We also found differences in the cytocidal effects of sterile water-induced hypotonic shock on RT112, T24, and J82 cells. RT112 cells were relatively more resistant to hypotonic shock, even when exposed to sterile water for 10 min. We analyzed the viability of DMSO- and NPPB-treated cells (at NPPB concentrations 50, 100, 200, and 300 µM) after exposure to sterile water. NPPB treatment decreased the viability of the bladder cancer cells upon exposure to sterile water to a greater extent than the reduction observed in DMSO-treated cells (proportions of viable DMSO- and NPPB-treated (50, 100, 200, and 300 µM) RT112 cells after 10 min of exposure to water: 9.1 ± 2.3%, 2.3 ± 0.7%, 1.7 ± 0.5%, 0.8 ± 0.5%, and 1.2 ± 1.2%, respectively). In the trypan blue-exclusion assay, we determined the proportion of live cells containing intact cell membranes by counting non-stained cells after exposure to sterile water (Figure 4). The trypan blue-exclusion assay showed results similar to those obtained using the MTT assay. The proportions of viable DMSO- and NPPB-treated (50, 100, 200, 300 µM) RT112 cells after 10 min of sterile water exposure were 13.6 ± 3.4%, 6.3 ± 1.2%, 2.0 ± 1.1%, 0.7 ± 0.7%, and 0%, respectively.
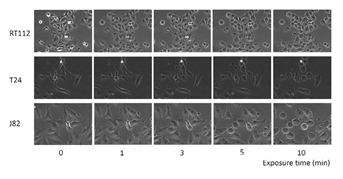
Figure 1: Representative images of RT112, T24, and J82 cells before and after exposure to sterile water.
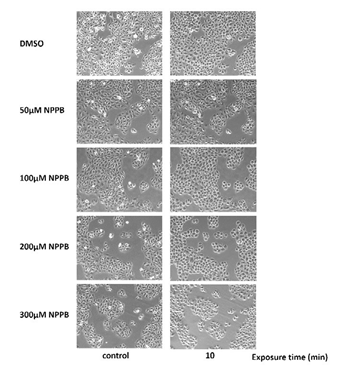
Figure 2: Representative images of DMSO- and NPPB-treated RT112 cells before and after exposure to sterile water. DMSO, dimethyl sulfoxide; NPPB, 5-nitro-2-(3-phenylpropylamino)-benzoic acid.

Figure 3: Viability of NPPB-treated (50, 100, 200, or 300 µM) bladder cancer cells after exposure to sterile water. NPPB, 5-nitro-2-(3-phenylpropylamino)-benzoic acid
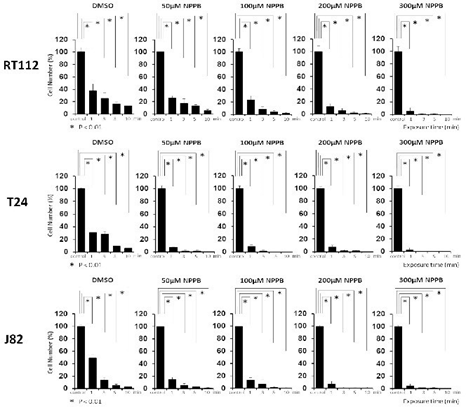
Figure 4: Cell membranes of NPPB-treated bladder cancer cells after exposure to sterile water. NPPB, 5-nitro-2-(3-phenylpropylamino)-benzoic acid.
Discussion
We previously demonstrated the cytocidal effects of sterile water on bladder cancer cells [9, 10]. However, the phenotypes differed between bladder cancer cell lines. Moreover, clinical research has demonstrated no significant differences in the recurrence-free rates between patients undergoing sterile water treatment and control patients [11]. Thus, treatment with sterile water alone may not be effective clinically. In the present study, we confirmed that treatment with a Cl- channel blocker enhanced the cytocidal effects of hypotonic shock on different bladder cancer cell lines. Thus, intravesical therapy using sterile water with a Cl- channel blocker may represent a feasible treatment strategy for patients with NMIBC, thereby avoiding radical surgery. Recent reports have discussed the roles of ion transporters in cancer cells; different ion transporters affect various organs in cancer patients [12-14]. For example, Cl- channels/ transporters play important roles in cancer cells, and the activation of Cl- channels is necessary for the regulation of cell volume of several gastric cancer cell lines. Iitaka et al. reported that NPPB treatment increased the cell volume by decreasing the regulatory volume and enhancing the cytocidal effects of the hypotonic solution in gastric cancer cell lines [15]. The inhibition of Cl- channels during hypotonic shock enhances cell swelling, thereby eliciting its cytocidal effects. Ion transport across the cell membrane represents the most efficient and rapid approach to altering cellular osmolarity [16]. Several studies have investigated the mechanisms underlying the regulation of cell volume upon exposing cells to abrupt changes in extracellular osmolarity. Cells exposed to hypotonic solutions initially swell, as observed by near-perfect osmometers, but approach the original cell volume based on regulatory cell volume decrease (RVD). Cells exposed to hypertonic solutions initially shrink, based on observation by near-perfect osmometers, but again, they approach the original cell volume owing to regulatory cell volume increase. Several ion transport systems are activated by cell swelling during RVD. RVD is caused by the outflow of water accompanied by the extracellular discharge of potassium chloride (KCl). K+ channel, Cl- channel, and K+/ Cl- co-transporters are the most frequently utilized transport systems for the release of KCl. Intracellular Cl- activity is important for the regulation of intracellular osmolarity, and it decreases during osmotic cell swelling. However, osmotic cell shrinkage is expected to increase the intracellular Cl- concentration. Thus, Cl- transport plays an important role in human cells. This study investigated whether treatment with a Cl- channel blocker enhanced the cytocidal effect of sterile water on bladder cancer cells. As in previous studies, the tested bladder cancer cell lines differed in their sensitivity to sterile water-induced hypotonic shock. RT112 cells demonstrated limited hypotonic swelling with reduced cell rupture. However, the cytocidal effect of sterile water was enhanced in these cells after treatment with the Cl- channel blocker, NPPB. RT112 cells treated with NPPB showed faster swelling and rupture upon exposure to sterile water than that observed in control cells. Moreover, the proportion of live cells after exposure to sterile water with NPPB decreased compared with that of the control cells. These findings provide experimental evidence that the combination of sterile water with a Cl- channel blocker was effective in enhancing the hypotonic shock-induced cytocidal effects on cultured bladder cancer cells that were originally resistant to sterile water. The high recurrence rate in NMIBC is attributed to the adhesion of free-floating tumor cells during TURBT. The most efficient period for introducing hypotonic treatment is early post-operative instillation because floating tumor cells have the greatest contact area with sterile water. We believe that single bladder irrigation directly after TURBT represents the most efficient treatment strategy. Thus, future studies should evaluate single bladder irrigation using a Cl- channel blocker and sterile water for 30 min each to achieve successful clinical application. To further understand the Cl- channel mechanisms in bladder cancer cells, we plan on designing future experiments using animal models and cells in which expression of Cl- channel is downregulated.
Conclusions
Blockade of the Cl- channel enhanced the cytocidal effects of sterile water-induced hypotonic shock in bladder cancer cells. However, this study has limitations. In particular, these findings must be validated using a patient cohort because specific characteristics of patient cancer cells and the immune system, relative to bladder cancer cell lines in vitro, may influence cancer cell survival. Nonetheless, intravesical therapy using sterile water after treatment with a Cl- channel blocker may represent a novel adjuvant therapy following
TURBT that is associated with high efficacy, low toxicity, and low cost. Moreover, this may represent a feasible therapeutic strategy for patients with NMIBC, potentially avoiding the need for radical surgery.
Funding:
None
Disclosure Statement: The authors report there are no competing interests to declare.
References
- Antoni S, Ferlay J, Soerjomataram I, et Bladder cancer incidence and mortality: A global overview and recent trends. Eur Urol 71 (2017): 96-108.
- Liedberg F, Hagberg O, Holmäng S, et Local recurrence and progression of non-muscle-invasive bladder cancer in Sweden: A population-based follow-up study. Scand J Urol 49 (2015): 290-295.
- Böhle A, Bock PR. Intravesical bacille Calmette-Guérin versus mitomycin C in superficial bladder cancer: Formal meta-analysis of comparative studies on tumor progression. Urology 63 (2004): 682-686.
- Sylvester RJ, van der Meijden APM, Lamm DL. Intravesical bacillus Calmette-Guerin reduces the risk of progression in patients with superficial bladder cancer: A meta-analysis of the published results of randomized clinical trials. J Urol 168 (2002): 1964-1970.
- Böhle A, Jocham D, Bock PR. Intravesical bacillus Calmette-Guerin versus mitomycin C for superficial bladder cancer: A formal meta-analysis of comparative studies on recurrence and toxicity. J Urol 169 (2003): 90-95.
- Järvinen R, Kaasinen E, Sankila A, et al. Long-term efficacy of maintenance bacillus Calmette-Guérin versus maintenance mitomycin C instillation therapy in frequently recurrent TaT1 tumours without carcinoma in situ: A subgroup analysis of the prospective, randomised FinnBladder I study with a 20-year follow-up. Eur Urol 56 (2009): 260-265.
- Sylvester RJ, Brausi MA, Kirkels WJ, et Long- term efficacy results of EORTC genito-urinary group randomized phase 3 study 30911 comparing intravesical instillations of epirubicin, bacillus Calmette-Guérin, and bacillus Calmette-Guérin plus isoniazid in patients with intermediate- and high-risk stage Ta T1 urothelial carcinoma of the bladder. Eur Urol 57 (2010): 766-773.
- Babjuk M, Burger M, Compérat EM, et al. European Association of Urology guidelines on non-muscle- invasive bladder cancer (TaT1 and carcinoma in situ) – 2019 update. Eur Urol 76 (2019): 639-657.
- Taoka R, Williams SB, Ho PL, et al. In-vitro cytocidal effect of water on bladder cancer cells: The potential role for intraperitoneal lavage during radical Can Urol Assoc J 9 (2015): 109-113.
- Matsuoka Y, Taoka R, Xia Z, et Hyperthermic therapy using warm sterile water enhances cytocidal effects on bladder cancer cells. Scand J Urol 54 (2020): 65-69.
- Sakai Y, Fujii Y, Hyochi N, et al. A large amount of distilled water ineffective for prevention of bladder cancer cell implantation at the time of transurethral resection. Hinyokika Kiyo 52 (2006): 173-175.
- Russell JM. Sodium-potassium-chloride cotransport. Physiol Rev 80 (2000): 211-276.
- Kunzelmann K. Ion channels and cancer. J Membr Biol 205 (2005): 159-173.
- Schönherr R. Clinical relevance of ion channels for diagnosis and therapy of cancer. J Membr Biol 205 (2005): 175-184.
- Iitaka D, Shiozaki A, Ichikawa D, et al. Blockade of chloride ion transport enhances the cytocidal effect of hypotonic solution in gastric cancer J Surg Res 176 (2012): 524-534.
- Lang F, Busch GL, Ritter M, et Functional significance of cell volume regulatory mechanisms. Physiol Rev 78 (1998): 247-306.